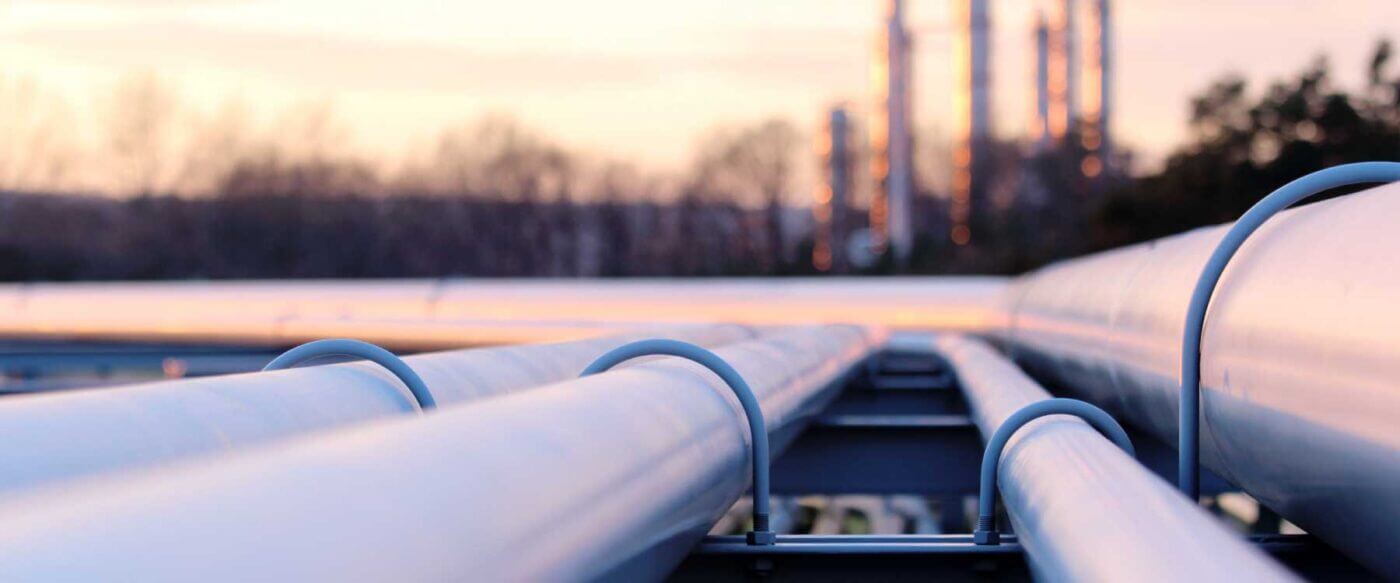
There’s a challenging road ahead for hydrogen imports to the EU
The European Commission has set ambitions targets as part of its REPowerEU plan to rapidly scale hydrogen production and import capacity, including a commitment to collectively produce — by 2030 — up to 10 million tonnes per year of domestic hydrogen and import a further 10 million tonnes per annum from potential export regions. Clean hydrogen deployment will be needed to decarbonise hard-to-abate sectors where hydrogen is being used as a feedstock to complete industrial processes (e.g., oil refining, fertilisers, and methanol production) and for nascent uses such as sustainable aviation fuels (SAF), maritime shipping, and greenfield steel.
To go from such targets to real-world deployment, there are many open questions to consider. In order to assess the suitability of different hydrogen transportation methods, CATF has conducted a first-of-a-kind analysis featured in the new report, Techno-Economic Realities of Long-Distance Hydrogen Transport, outlining the cost of importing hydrogen to Europe’s largest seaport, the Port of Rotterdam in the Netherlands, from potential future hydrogen exporters. The modelling, which was conducted by the major engineering firm KBR Inc., includes four different transportation pathways:
- (1) Pipeline transport of gaseous hydrogen from Algeria and Norway
- Maritime transport of either (2) liquid hydrogen, (3) cracked and uncracked ammonia1, or (4) liquid organic hydrogen carriers (LOHC) from Norway and the Arabian Gulf, as well as North and South America
The study focused on hydrogen produced with natural gas and carbon capture, what’s more commonly known as ‘low-carbon’ or ‘blue’ hydrogen. This option was chosen because low-carbon hydrogen can scale more rapidly than electrolytic hydrogen (e.g., renewable hydrogen), which requires abundant zero-carbon electricity, and so the modelling in the report would be most relevant to decision makers in the near term. However, the calculated costs to export, transport, and import hydrogen should also apply to hydrogen produced via any other means.
Here’s what we found:
- Due to hydrogen’s physical properties, particularly its low volumetric energy density, importing the molecule over long distances will be expensive and relatively inefficient no matter by ship or pipeline;
- Hydrogen transported by liquid carriers like ammonia incur a significant energy penalty — and thus cost penalty — at the point of import when liberated (ammonia cracking) that will likely stay significant even with scale and technical improvements;
- Between transporting hydrogen via ship or pipeline, pipeline transport consistently ranks as the most cost-effective option in the short and long term; and
- The transport of “uncracked” ammonia rather than pure hydrogen offers the cheapest pathway for transporting hydrogen molecules, beating even pipeline delivery of pure gaseous hydrogen although the product delivered is different.
See the figure below for a detailed breakdown of the levelized cost of hydrogen for the aforementioned pathways.
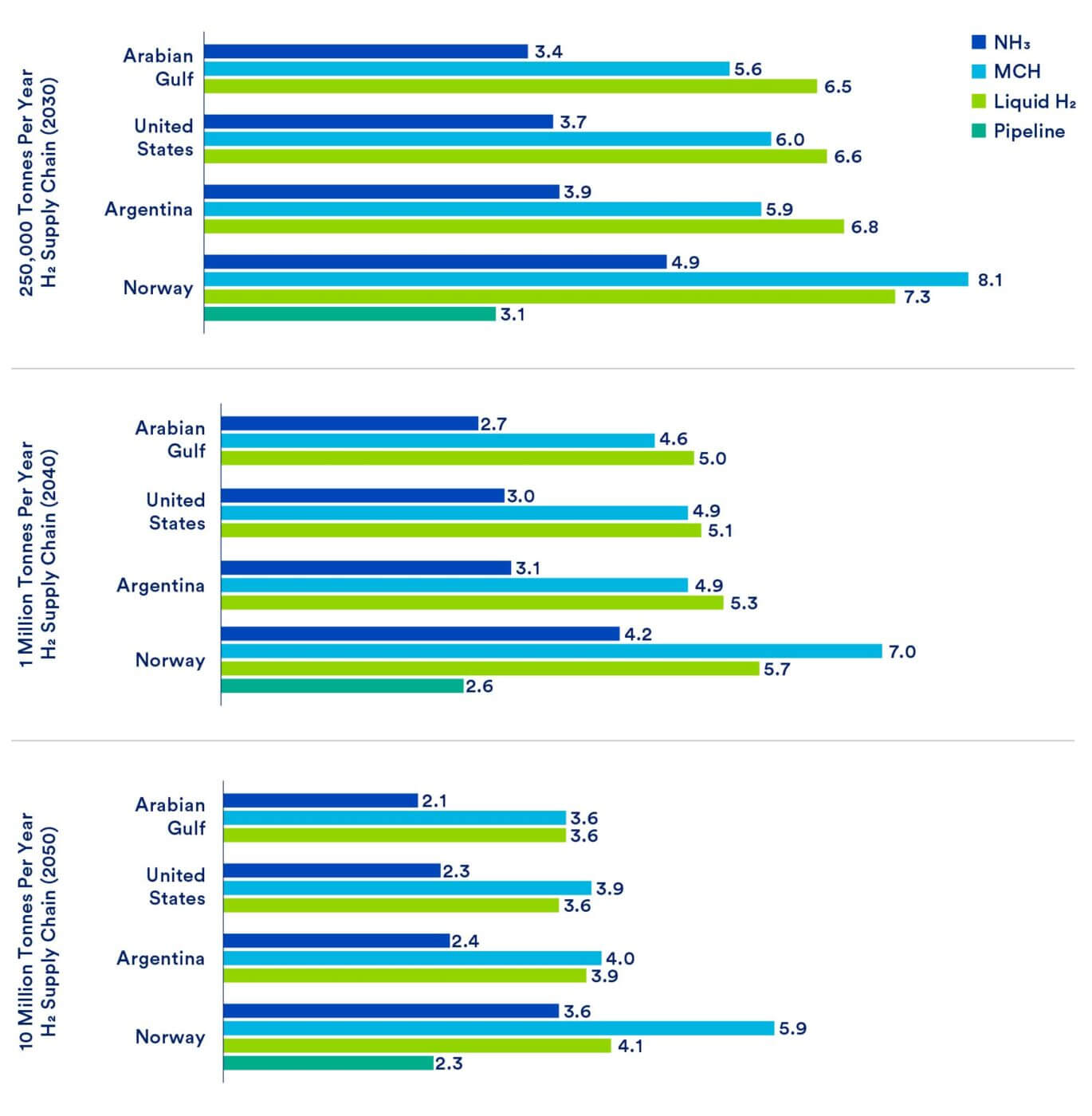
Policy Implications
These results show that transporting low-carbon hydrogen by sea presents significant hurdles due to hydrogen’s inherent physical properties. Given the enormity of the challenge, public policy must be carefully designed to prioritise the most cost-effective technologies and to prevent spending public funding on expensive infrastructure investments that could lead to inefficient and ineffective methods of decarbonisation.
Here’s what it means for policy:
- Europe should re-examine its hydrogen demand forecasts to develop realistic estimates. At present, some prominent policy targets, such as the EU’s goal of supplying the European market with 20 million tonnes per year of clean hydrogen by 2030, 10 million of which would come from domestic production and the remaining 10 million would come from imports, remain challenging and should be reassessed. Moreover, by focusing on this very near-term goal, European governments risk missing opportunities to integrate the broader set of climate-friendly technologies that will be needed to achieve net zero by midcentury.
- The EU’s plans for hydrogen deployment should focus on “no-regrets” sectors, where no other energy-efficient or cost-effective decarbonisation options are currently available. Hydrogen “no-regrets” sectors include those that already use hydrogen as a chemical feedstock today, such as ammonia production, methanol, and refining, as well as those that may be commercially impossible to decarbonise without clean hydrogen in the future, such as heavy-duty transportation (trucking, aviation, shipping) and greenfield (primary) steel.
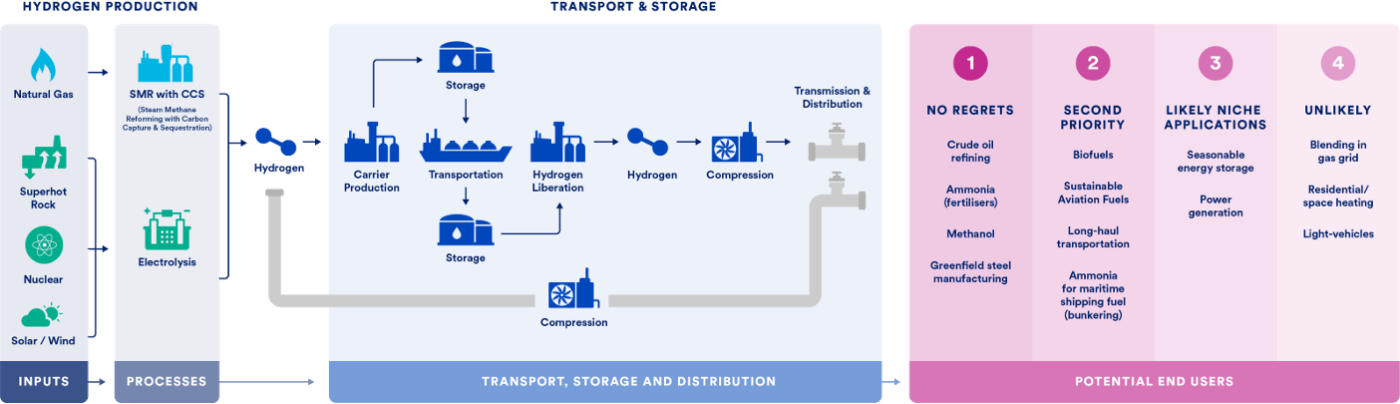
- Deployment of Liquid Organic Hydrogen Carriers (LOHC) or Liquefied Hydrogen imports should be limited to niche applications. Policymakers need to carefully examine investments in LOHC and liquid hydrogen-based supply chains. Figure 3 provides a snapshot of the infrastructure capital costs and the levelized cost of importing 10 million tonnes per year of hydrogen from the Arabian Gulf. Both the LOHC and liquid hydrogen pathways require twice the capital investment compared to importing and cracking ammonia, which translates to almost double the levelized cost as well. Given these significantly higher deployment costs, both LOHC and liquid hydrogen should be limited to niche applications.
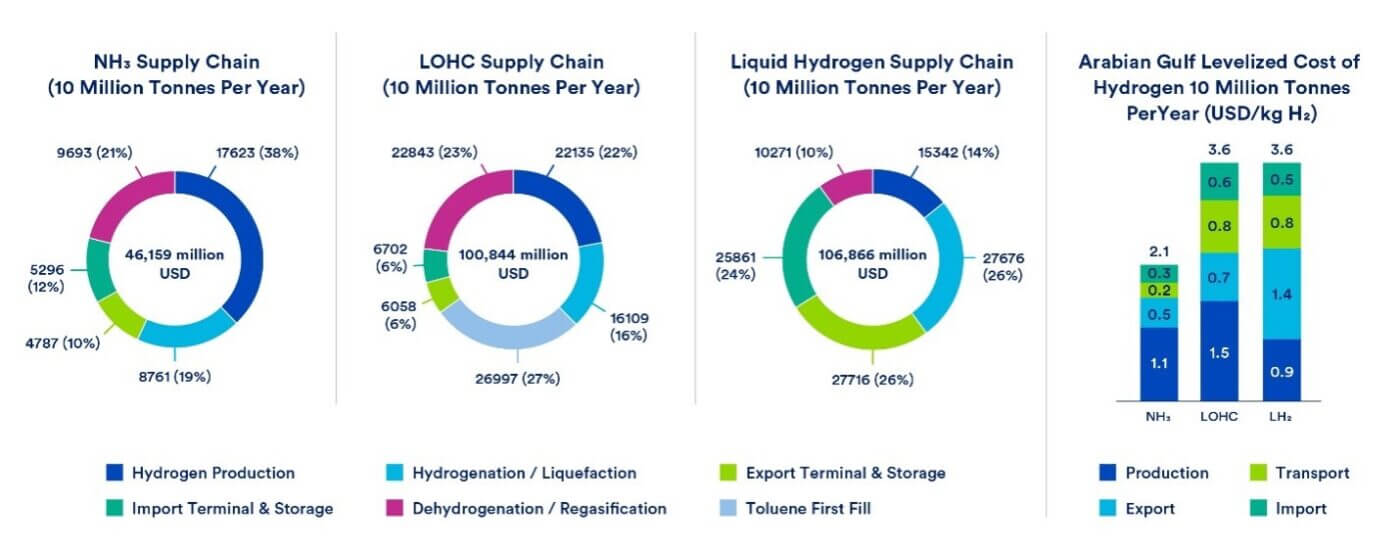
- The world needs an internationally recognised system to certify clean hydrogen for imports to be a viable hydrogen procurement strategy anywhere. Although several international groups and national governments are working to develop methodologies for certifying clean hydrogen and ammonia, the lack of clear standards and an internationally recognised certification system remains an important barrier to investment in nascent hydrogen markets.
- Further work is needed to understand the infrastructure needs and cost associated with building out storage and distribution systems for delivering hydrogen to end users. Hydrogen will have to be delivered in a reliable and cost-effective manner to end users. The ‘last-mile’ costs and challenges are potentially significant and merit the attention from policymakers.
- Transporting liquefied natural gas (LNG) to Europe and using it to produce hydrogen at the point of import (with low-carbon energy inputs, strict methane emissions control, and carbon capture and storage) will likely be more feasible and cost-effective than importing low-carbon hydrogen from distant suppliers. This option was not considered in this study but bears further exploration, especially since EU countries are currently building out an extensive LNG importing infrastructure following the disruption to European gas markets in early 2022.
A robust approach to the climate challenge will utilise the advantages of a variety of technology pathways, including hydrogen. However, European policymakers must think realistically about viable domestic production and import pathways for hydrogen and follow a technology open approach to scaling hydrogen based on their merits of emissions reduction, technical feasibility, and cost-effectiveness.
More broadly, resources should be leveraged to prioritise the most promising and cost-effective technologies first, recognising that, while having options is important, so is avoiding expensive investments in infrastructure that are inherently inefficient or unlikely to be used. The reality is that additional technologies and a deeper paradigm change will be needed to achieve emissions neutrality in Europe.
1 Due to the challenges presented hydrogen’s physical properties, it requires conversion into a carrier for transportation via maritime shipping. Ammonia is among the most promising hydrogen carriers, however, it necessitates the release of the hydrogen molecule through a process known as ‘ammonia cracking’ upon arrival at a port. This dehydrogenation process demands substantial energy inputs. To account for this requirement, the study also considers a scenario where ammonia is transported without dehydrogenation (uncracked ammonia) for direct use in applications such as bunkering fuel or fertiliser production.