Unlocking Europe’s CO2 Storage Potential
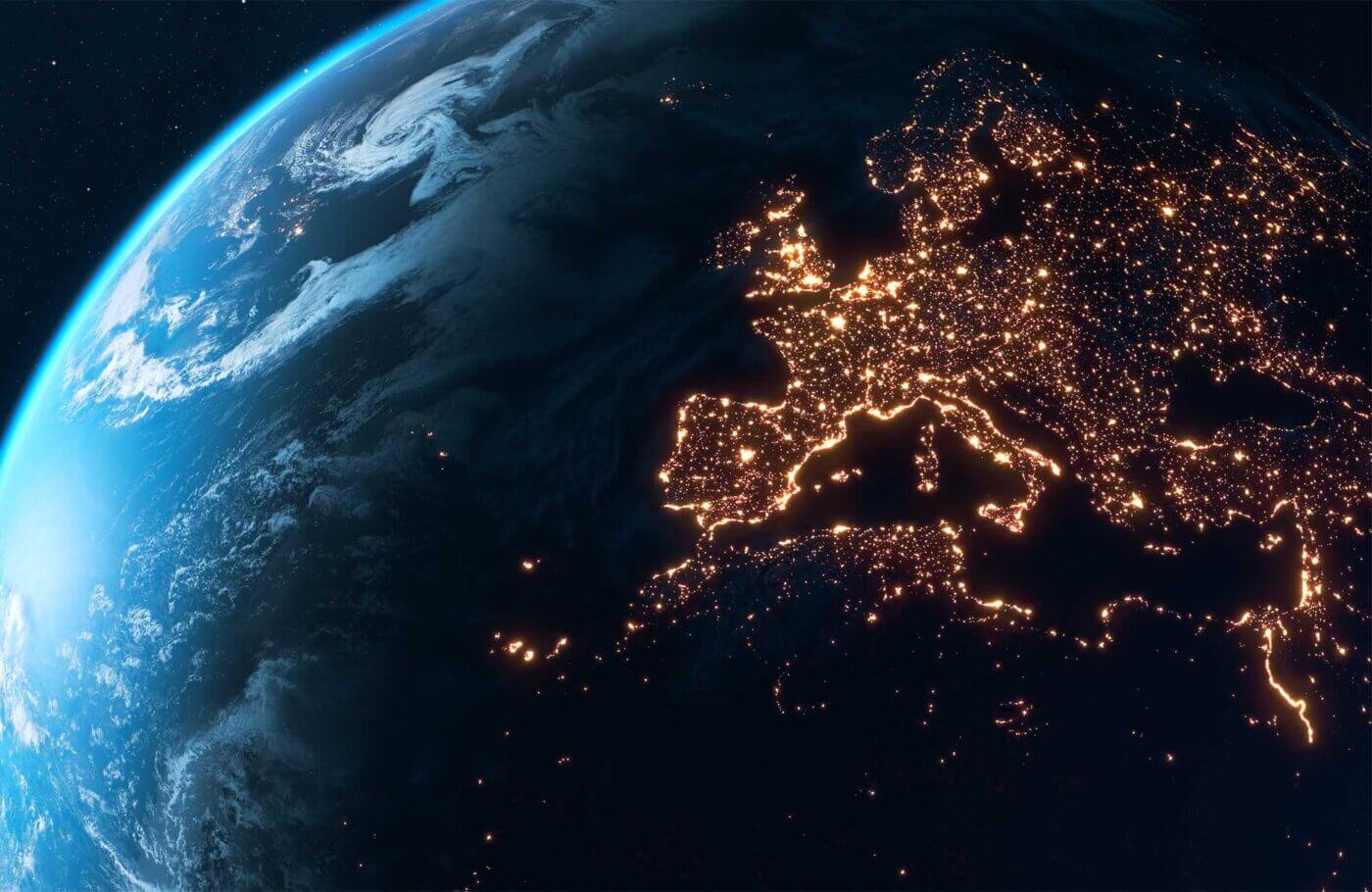
About this report
Carbon capture and storage (CCS) is widely recognised as a key part of reaching net zero especially as a solution for sectors with emissions that are hard to abate or for carbon removal technologies. A key barrier hindering large-scale uptake of CCS is the limited information on the geographical availability of CO2 storage.
This goal of this study, commissioned by CATF and prepared by Element Energy, was to provide a high-level estimation of the distribution and capacity of CO2 storage reservoirs across Europe and link these to where emissions from potential CCS-adopting industries are located. It aims to help policy makers understand where support of CCS development is likely to be most impactful and help investors and project developers understand the most promising regions for investment in CO2 transport and storage.
About the Authors
This report has been prepared by Element Energy, an ERM Group company.
Element Energy is a strategic energy consultancy, specialising in the intelligent analysis of low carbon energy. The team of over 100 specialists provides consultancy services across a wide range of sectors, including the built environment, carbon capture and storage, industrial decarbonisation, smart electricity and gas networks, energy storage, renewable energy systems and low carbon transport. Element Energy provides insights on both technical and strategic issues, believing that the technical and engineering understanding of the real world challenges support the strategic work. In June 2021, Element Energy joined the ERM Group, the largest independent sustainability consultancy, with a global footprint and over 7,000 employees worldwide.
This work was commissioned by Clean Air Task Force (CATF).
Clean Air Task Force (CATF) is a global nonprofit organization working to safeguard against the worst impacts of climate change by catalysing the rapid development and deployment of low-carbon energy and other climate-protecting technologies. With 25 years of internationally recognized expertise on climate policy and a fierce commitment to exploring all potential solutions, CATF is a pragmatic, non-ideological advocacy group with the bold ideas needed to address climate change. CATF has offices in Boston, Washington D.C., and Brussels, with staff working virtually around the world.
Authors
Silvian Baltac, Associate Partner
Elian Pusceddu, Principal Consultant
Conor O’Sullivan, Senior Consultant
Hannah Galbraith-Olive, Consultant
Cameron Henderson, Consultant
For comments or queries, please contact the authors at: [email protected]
Disclaimer
This study was commissioned by CATF. The conclusions and recommendations do not necessarily represent the view of CATF. Whilst every effort has been made to ensure the accuracy of this report, neither CATF nor Element Energy warrant its accuracy or will, regardless of its or their negligence, assume liability for any foreseeable or unforeseeable use made of this report which liability is hereby excluded.
Introduction
Carbon capture is likely to be a key technology for decarbonising industry, but is there enough geological storage capacity across Europe?
Context
- Carbon capture and storage (CCS) is widely recognised as a key part of reaching net zero especially as a solution for sectors with emissions that are hard to abate or for carbon removal technologies.
- CCS is likely to be a key technology for decarbonising industry, particularly in retrofit applications where currently operational facilities can be decarbonised without changes to the underlying process.
- A key barrier hindering large-scale uptake of CCS is the limited information on the geographical availability of CO2 storage.
Purpose
- The aim of this study was to provide a high-level estimation, in both 2035 and 2050, of the distribution and capacity of CO2 storage reservoirs across Europe and link these to where emissions from potential CCS-adopting industries are located.
- The result of this is to identify opportunities to optimise the storage of large quantities of emissions in regions with high geological CO2 storage capacity.
- This study aims to help policy makers understand where support of CCS development is likely to be most impactful, such as regions of high emissions which could particularly benefit from faster CO2 transport and storage development.
- It will also help investors and project developers understand the most promising regions for investment in CO2 transport and storage.
Key considerations and uncertainties when considering the interaction between large scale emitters and CO2 storage sites
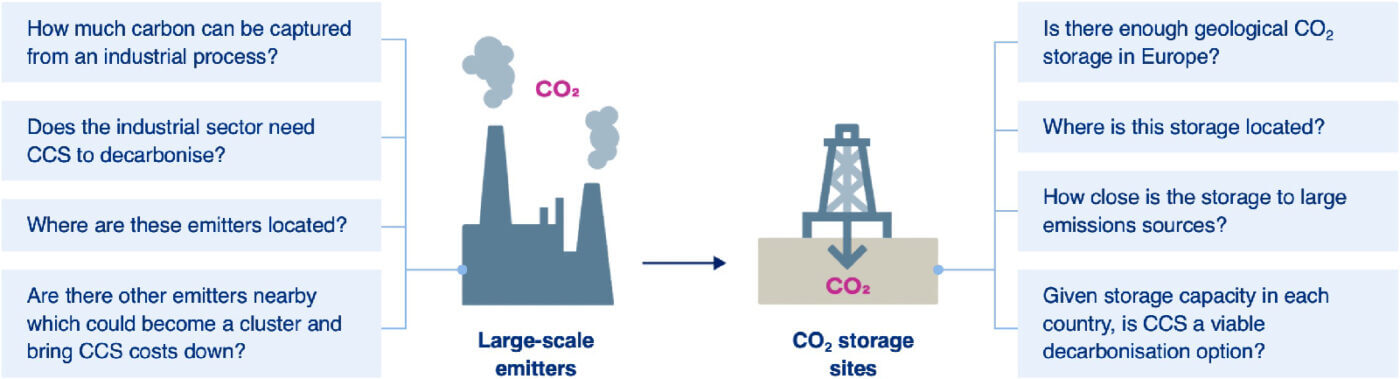
The project overlays potential CCS demand and CO2 storage capacity estimates to identify optimal CO2 transport and storage development across Europe
Our approach
Our analysis consisted of a literature review and numerical analysis to understand the optimal CO2 transport and storage development across Europe, and how further decisions by policymakers and the private sectors should try to leverage the full geologic potential of Europe.
As part of this:
- A Domestic scenario (widespread storage) and an Export scenario (storage limited to a few key basins) were developed where low/high storage capacities are considered at a country level.
- A database of current industrial emissions (IEP) was used to develop a Technical Potential scenario (to test the limits of storage capacities) and a Prioritised CCS scenario (linked to the upper end of literature estimates for CCS demand) for both 2035 and 2050. Non-industrial CCS demand was considered independently.
- Source-to-sink matching of the supply and demand for CCS lead to the development of CO2 transport and storage scenarios in 2035 and 2050.
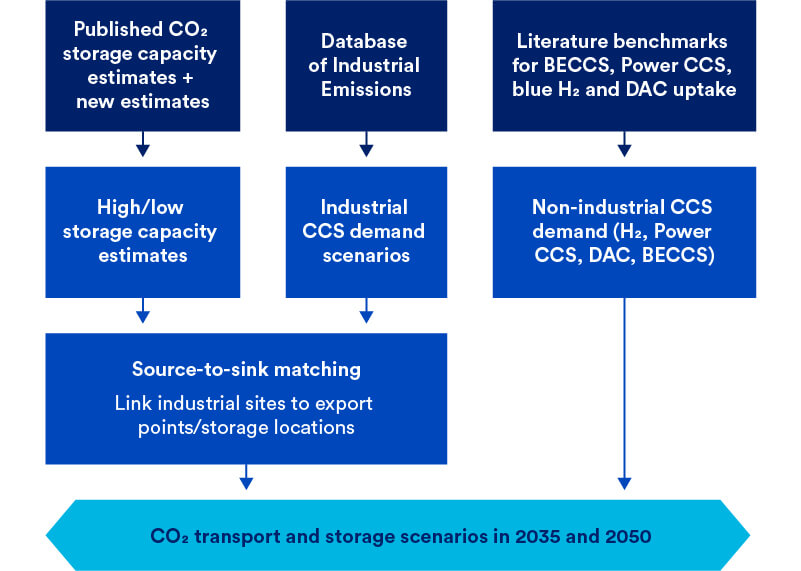
Understanding European Storage Capacity
European estimates of CO2 storage capacity form two scenarios – an Export scenario with key storage basins, and a Domestic scenario with widespread storage.
CO2 storage capacity estimates
- High and low CO2 storage capacity estimates were derived from a wide range of published literature assessing country-level CO2 storage capacity.
- Additional bottom-up estimates were made for countries where only one estimate was found in the literature. These are new estimates based on available geological data.
Export Scenario
- In the export scenario, only a few key storage basins are developed.
- These basins are the North Sea, East Irish Sea, Aquitaine Basin, Adriatic Sea, Aegean Sea and the Black Sea.
- Export locations are assigned based on announced projects and, if needed, demand from emitters.
- Beyond the announced projects, these export points represent a means of aggregating national or sub-national areas of captured emissions.
- The scenario developed in this study is illustrative – in reality, there could be many more export points developed, especially along coastlines.
Domestic Scenario
- In the domestic storage scenario, many more, widespread storage sites are considered.
- All countries with sufficient geological storage capacity (≥1 GtCO2 high storage capacity estimate) are assigned a storage location.
- Some countries are assigned multiple storage locations to reflect the broader distribution of sedimentary basins.
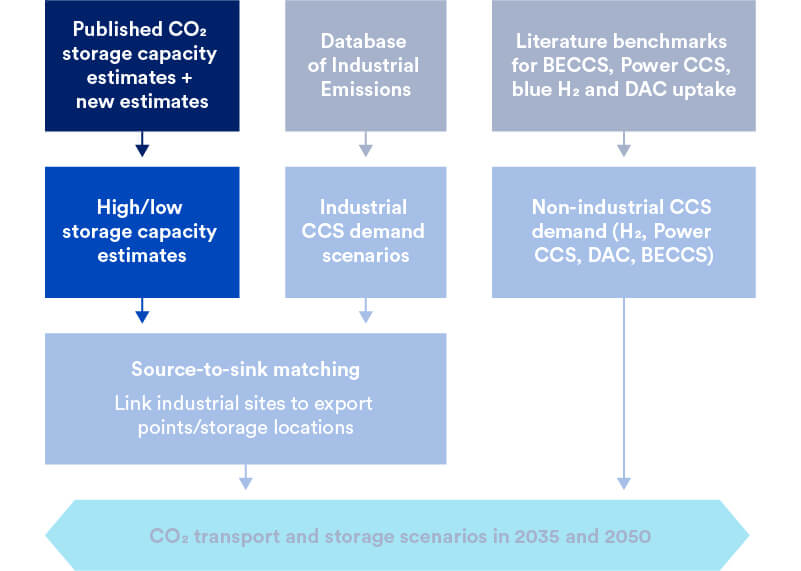
Theoretical CO2 storage capacity across Europe is estimated to be between 262 and 1520 GtCO2
This study identifies 17 countries that could potentially store over 10 GtCO2.
- Bulgaria (3-450 GtCO2)1 and Norway (70-280 GtCO2)2 have the largest theoretical storage capacities.
- Belgium, Austria and Slovenia have the smallest theoretical storage capacities (≤1 GtCO2) which may be a barrier to CCS in these countries.
- Finland and Estonia have no suitable sedimentary basins for CO2 storage.
High and low storage capacity estimates were made for each country.
- Estimates were compiled from across the literature.
- These estimates were supplemented with estimates for countries with only one capacity estimate in the literature.3
Additional subsurface information will be required to identify the precise location of CO2 injection.
- Storage will not be possible in every location of every sedimentary basin. Some locations will be more suitable than others based on their subsurface geology.
- In order to know the exact location of where CO2 injection into the subsurface might be in the future, a better understanding of the subsurface is required, particularly in regions outside the North Sea.
- Other regions may not be suitable for CO2 storage for different reasons – for example, Italy has a high risk of earthquakes which may pose a risk to CO2 storage in that sedimentary basin.4
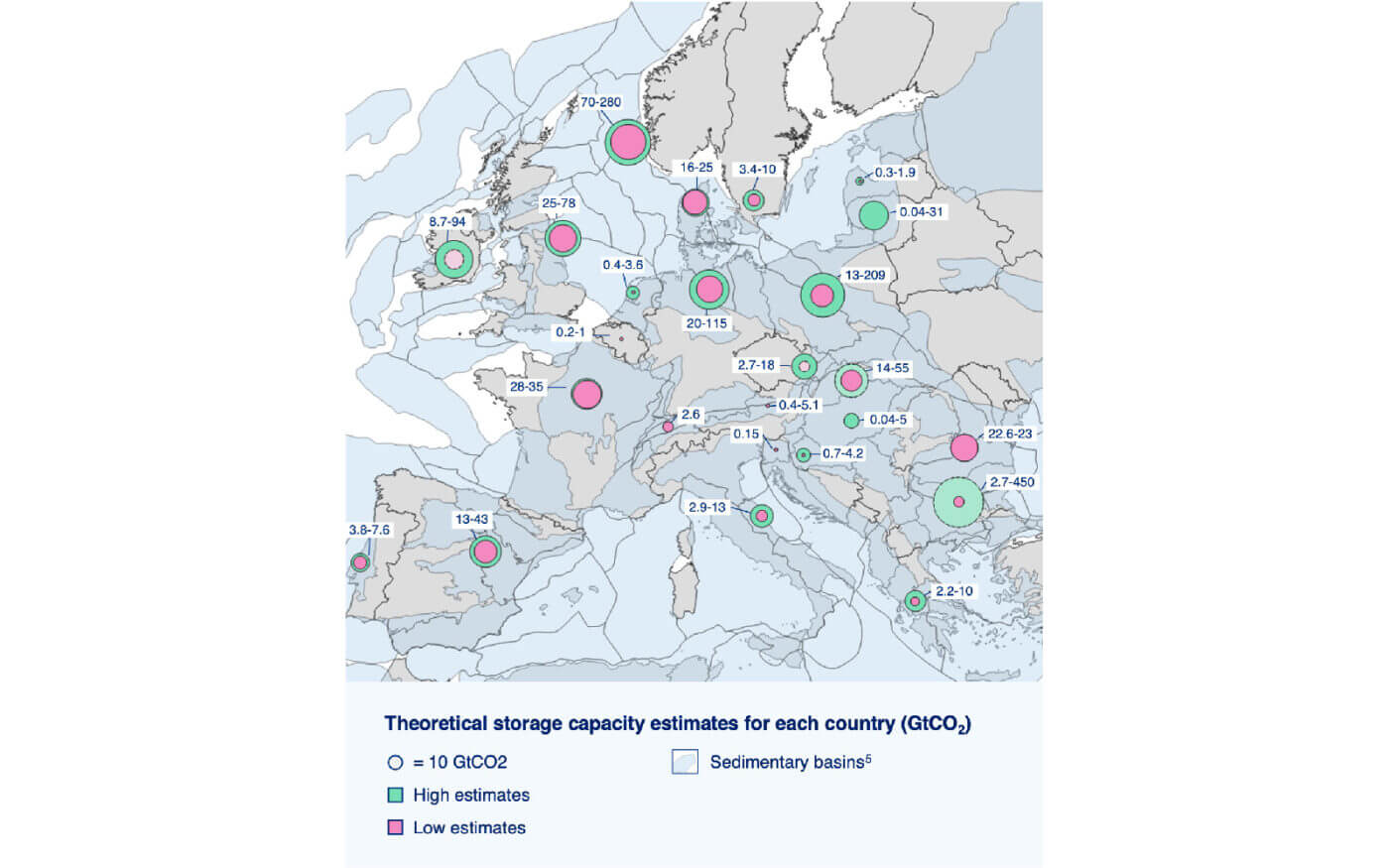
Footnotes
- Bottom-up estimates were made for Bulgaria’s storage capacity (more speculative)
- Storage capacities for Norway are based on published literature (more reliable)
- Bottom-up estimates were made for Ireland (low estimate), Czechia (low estimate), Slovakia (high estimate) and Bulgaria (high estimate)
- Storage Capacity in the West Mediterranean
- CGG Robertson Basins and Plays
Credits
About the Authors
This report has been prepared by Element Energy, an ERM Group company.
Element Energy is a strategic energy consultancy, specialising in the intelligent analysis of low carbon energy. The team of over 100 specialists provides consultancy services across a wide range of sectors, including the built environment, carbon capture and storage, industrial decarbonisation, smart electricity and gas networks, energy storage, renewable energy systems and low carbon transport. Element Energy provides insights on both technical and strategic issues, believing that the technical and engineering understanding of the real world challenges support the strategic work. In June 2021, Element Energy joined the ERM Group, the largest independent sustainability consultancy, with a global footprint and over 7,000 employees worldwide.
This work was commissioned by Clean Air Task Force (CATF).
Clean Air Task Force (CATF) is a global nonprofit organization working to safeguard against the worst impacts of climate change by catalysing the rapid development and deployment of low-carbon energy and other climate-protecting technologies. With 25 years of internationally recognized expertise on climate policy and a fierce commitment to exploring all potential solutions, CATF is a pragmatic, non-ideological advocacy group with the bold ideas needed to address climate change. CATF has offices in Boston, Washington D.C., and Brussels, with staff working virtually around the world.
Authors
Silvian Baltac, Associate Partner
Elian Pusceddu, Principal Consultant
Conor O’Sullivan, Senior Consultant
Hannah Galbraith-Olive, Consultant
Cameron Henderson, Consultant
For comments or queries, please contact the authors at: [email protected]
Disclaimer
This study was commissioned by CATF. The conclusions and recommendations do not necessarily represent the view of CATF. Whilst every effort has been made to ensure the accuracy of this report, neither CATF nor Element Energy warrant its accuracy or will, regardless of its or their negligence, assume liability for any foreseeable or unforeseeable use made of this report which liability is hereby excluded.
Future operational CO2 storage capacity is likely to be a fraction of theoretical and effective storage capacities presented in this study
Storage capacity estimates presented in this study will be refined in the future as countries gain a greater understanding of their subsurface. The estimates presented in this study refer to either the theoretical capacity or effective capacity in the resource pyramid for CO2 storage capacity.
In this study, each literature estimates are categorised into three levels:
- Country-level estimates are estimates where no methodology is publicly available although a high-level storage capacity value is published for the entire country – these are assumed to be theoretical storage capacities.
- Basin-level estimates are those which use regional aquifers within a country to estimate CO2 storage capacity –these are theoretical storage capacities.
- Trap-level estimates are those which consider the location of geological traps under which CO2 can be stored – these are effective storage capacities.*
As countries gain a greater understanding of their subsurface, theoretical storage capacity estimates will become effective and practical capacity estimates, which may reduce the overall European storage capacity presented in this study.
Only a fraction of theoretical storage capacity will be commercially developed for a variety of technical, economic, legal and social reasons.
- The exact fraction of geological storage capacity that will become commercially operational is highly site specific.
- This fraction will also likely be determined by regulatory barriers applied at a European or country-level.
- These restrictions may vary depending on the demand for CO2 storage in that region as well as the availability of more suitable storage areas nearby.
Resource pyramid for CO2 storage capacity1
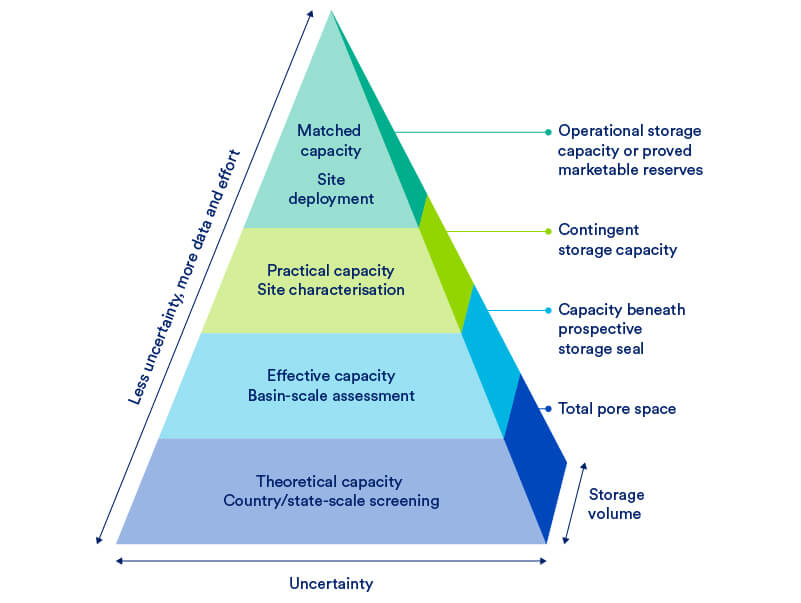
Footnotes
*Traps are geological features where CO2 can be stored. The reservoir rock layer which contains CO2 must be overlain by a seal (e.g., a cap rock which does not let fluid pass through it) to ensure the CO2 is permanently stored.
1. (PDF) Carbon sequestration potential of the South Wales Coalfield (researchgate.net)
Credits
About the Authors
This report has been prepared by Element Energy, an ERM Group company.
Element Energy is a strategic energy consultancy, specialising in the intelligent analysis of low carbon energy. The team of over 100 specialists provides consultancy services across a wide range of sectors, including the built environment, carbon capture and storage, industrial decarbonisation, smart electricity and gas networks, energy storage, renewable energy systems and low carbon transport. Element Energy provides insights on both technical and strategic issues, believing that the technical and engineering understanding of the real world challenges support the strategic work. In June 2021, Element Energy joined the ERM Group, the largest independent sustainability consultancy, with a global footprint and over 7,000 employees worldwide.
This work was commissioned by Clean Air Task Force (CATF).
Clean Air Task Force (CATF) is a global nonprofit organization working to safeguard against the worst impacts of climate change by catalysing the rapid development and deployment of low-carbon energy and other climate-protecting technologies. With 25 years of internationally recognized expertise on climate policy and a fierce commitment to exploring all potential solutions, CATF is a pragmatic, non-ideological advocacy group with the bold ideas needed to address climate change. CATF has offices in Boston, Washington D.C., and Brussels, with staff working virtually around the world.
Authors
Silvian Baltac, Associate Partner
Elian Pusceddu, Principal Consultant
Conor O’Sullivan, Senior Consultant
Hannah Galbraith-Olive, Consultant
Cameron Henderson, Consultant
For comments or queries, please contact the authors at: [email protected]
Disclaimer
This study was commissioned by CATF. The conclusions and recommendations do not necessarily represent the view of CATF. Whilst every effort has been made to ensure the accuracy of this report, neither CATF nor Element Energy warrant its accuracy or will, regardless of its or their negligence, assume liability for any foreseeable or unforeseeable use made of this report which liability is hereby excluded.
Literature estimates of geological storage capacity vary in reliability – trap-level estimates are likely to be the most reliable
For each country-wide geological storage capacity estimate analysed in the publicly-available literature, different levels of detail are presented.
Those estimates which use known geological traps* (i.e., trap-level, under which CO2 can be stored permanently) to calculate storage capacity are likely to be the most reliable as the location and extent of the storage location is known. However, these capacity estimates are limited to known trap locations.
For basin-level and country-level storage capacity estimates, the fraction of the basin which will contain geological traps under which CO2 can be stored is estimated as part of a variable known as the storage efficiency factor. Therefore, these estimates are less reliable.
- CO2 storage capacity (graph to the right) is greatest in Bulgaria, Norway, Poland and Germany.
- Estonia and Finland both do not have suitable geological formations for CO2 storage in sedimentary basins.
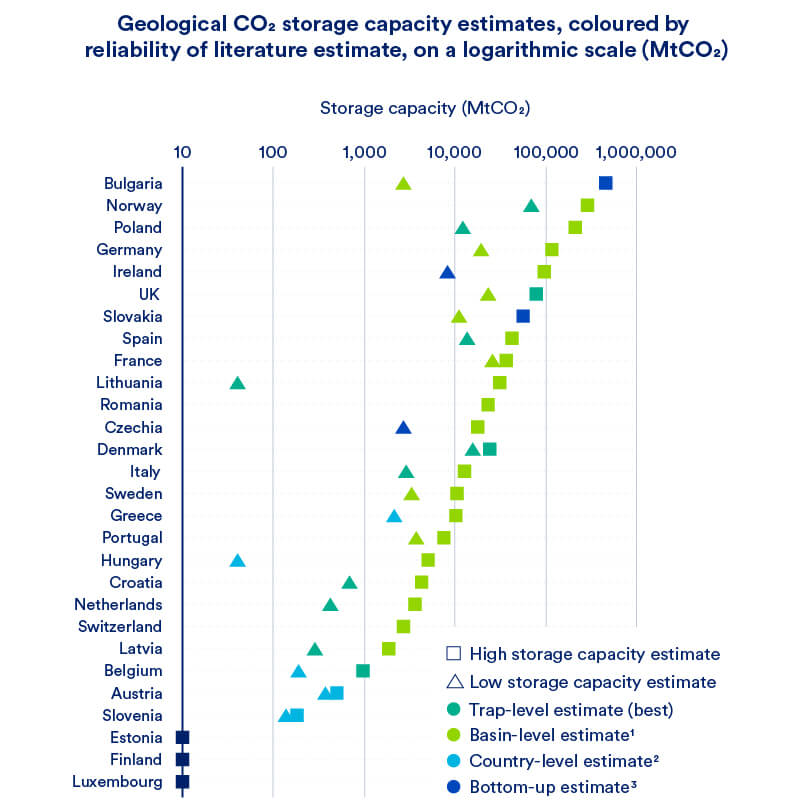
Footnotes
*Traps are geological features where CO2 can be stored. The reservoir rock layer which contains CO2 must be overlain by a seal (e.g., a cap rock which does not let fluid pass through it) to ensure the CO2 is permanently stored.
1. Basin level estimates are those which use regional aquifers within a country to estimate CO2 storage capacity.
2. Country-level estimates are estimates where no methodology is publicly available although the storage capacity value is published for the entire country.
3. Storage capacity estimates made as part of this study.
Credits
About the Authors
This report has been prepared by Element Energy, an ERM Group company.
Element Energy is a strategic energy consultancy, specialising in the intelligent analysis of low carbon energy. The team of over 100 specialists provides consultancy services across a wide range of sectors, including the built environment, carbon capture and storage, industrial decarbonisation, smart electricity and gas networks, energy storage, renewable energy systems and low carbon transport. Element Energy provides insights on both technical and strategic issues, believing that the technical and engineering understanding of the real world challenges support the strategic work. In June 2021, Element Energy joined the ERM Group, the largest independent sustainability consultancy, with a global footprint and over 7,000 employees worldwide.
This work was commissioned by Clean Air Task Force (CATF).
Clean Air Task Force (CATF) is a global nonprofit organization working to safeguard against the worst impacts of climate change by catalysing the rapid development and deployment of low-carbon energy and other climate-protecting technologies. With 25 years of internationally recognized expertise on climate policy and a fierce commitment to exploring all potential solutions, CATF is a pragmatic, non-ideological advocacy group with the bold ideas needed to address climate change. CATF has offices in Boston, Washington D.C., and Brussels, with staff working virtually around the world.
Authors
Silvian Baltac, Associate Partner
Elian Pusceddu, Principal Consultant
Conor O’Sullivan, Senior Consultant
Hannah Galbraith-Olive, Consultant
Cameron Henderson, Consultant
For comments or queries, please contact the authors at: [email protected]
Disclaimer
This study was commissioned by CATF. The conclusions and recommendations do not necessarily represent the view of CATF. Whilst every effort has been made to ensure the accuracy of this report, neither CATF nor Element Energy warrant its accuracy or will, regardless of its or their negligence, assume liability for any foreseeable or unforeseeable use made of this report which liability is hereby excluded.
The Domestic scenario represents widespread development of CO2 storage locations
The study identifies illustrative storage locations where CO2 injection may occur.
- The announced storage locations (blue discs) are based on both announced1 and pilot projects2 – assumed to be developed in 2035.
- These locations are where CO2 is injected into the subsurface, either onshore or from where it is transported for offshore injection.
- If a region does not have either an announced or pilot project, the storage location is reflective of the broader distribution of sedimentary basins in that region or at the nearest onshore location (purple discs). These sites are assumed to be developed by 2050.
- Belgium, Austria and Slovenia all have a country-level high storage capacity estimate ≤1GtCO2 so were not assigned their own storage location.
- These do not represent actual storage locations, but act as a location where national or sub-national emissions can be aggregated for storage.
- If a country has more than one storage location, each storage location has access to a representative fraction of the country’s geological storage*.
Additional or alternative storage sites may be established.
- The scenario developed in this study is illustrative – in reality, there could be more (or fewer) storage sites developed.
- While the sites identified are plausible locations, the exact location of future injection will depend on a host of technical and economic factors, including demand for CCS. Thus, it could be economic to develop a CO2 storage site in closer proximity to emitters, given suitable geology.
- No distinction has been made between onshore/offshore CO2 storage locations, even though there may be strong political and economic drivers which will influence the location of CO2 storage.
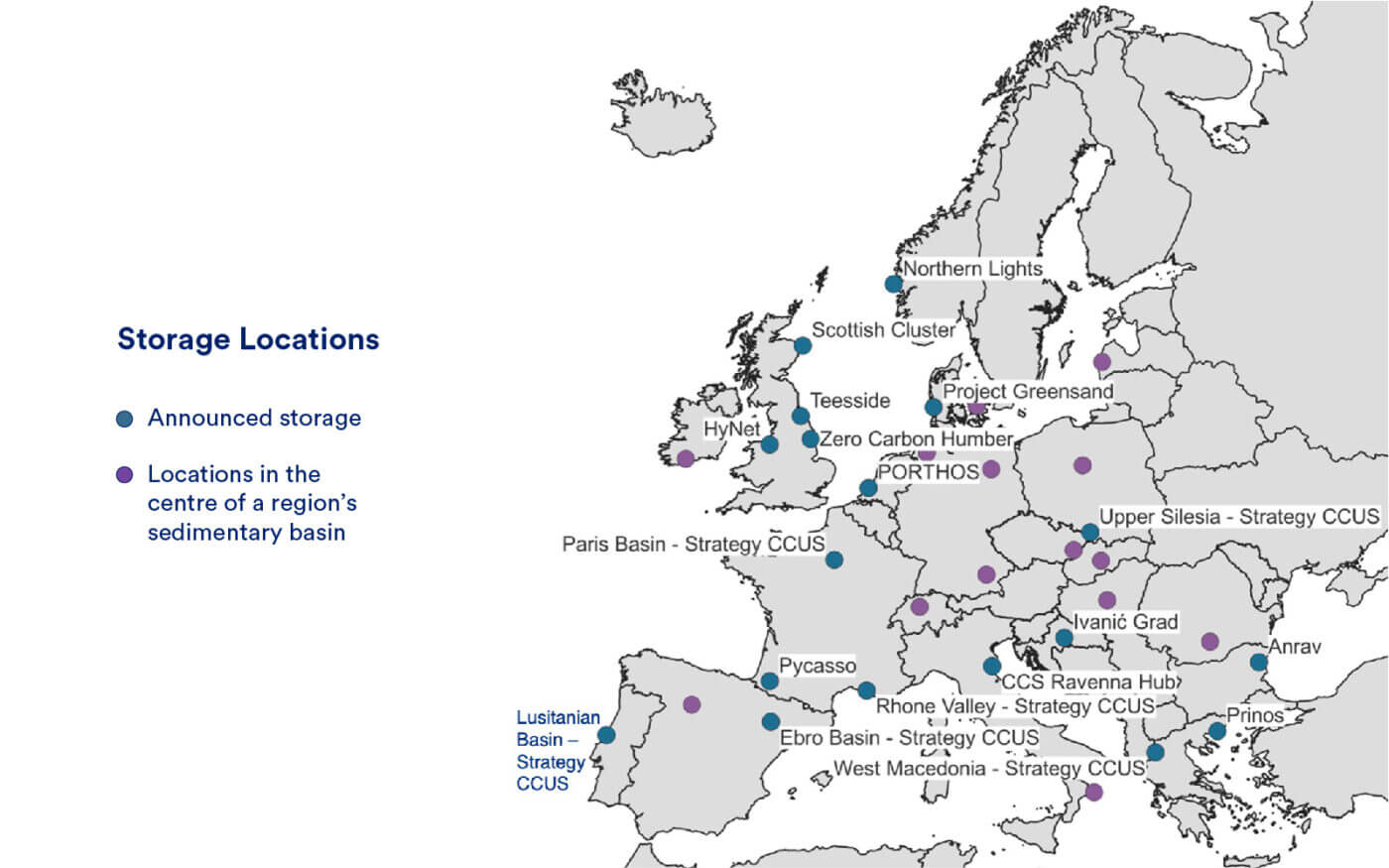
Footnotes
- Based on Europe Carbon Capture Project Map – Clean Air Task Force (catf.us)
- Mapping potential (WP2) | Strategy CCUS – these pilot locations are not active test sites, rather promising areas identified for further development. Since the storage potential in Romania is more in the centre/south-west of the country, the Strategy CCUS location was replaced by a point representative of Romanian sedimentary basins.
*Further detail on the fractions used can be found in the Appendix
Credits
About the Authors
This report has been prepared by Element Energy, an ERM Group company.
Element Energy is a strategic energy consultancy, specialising in the intelligent analysis of low carbon energy. The team of over 100 specialists provides consultancy services across a wide range of sectors, including the built environment, carbon capture and storage, industrial decarbonisation, smart electricity and gas networks, energy storage, renewable energy systems and low carbon transport. Element Energy provides insights on both technical and strategic issues, believing that the technical and engineering understanding of the real world challenges support the strategic work. In June 2021, Element Energy joined the ERM Group, the largest independent sustainability consultancy, with a global footprint and over 7,000 employees worldwide.
This work was commissioned by Clean Air Task Force (CATF).
Clean Air Task Force (CATF) is a global nonprofit organization working to safeguard against the worst impacts of climate change by catalysing the rapid development and deployment of low-carbon energy and other climate-protecting technologies. With 25 years of internationally recognized expertise on climate policy and a fierce commitment to exploring all potential solutions, CATF is a pragmatic, non-ideological advocacy group with the bold ideas needed to address climate change. CATF has offices in Boston, Washington D.C., and Brussels, with staff working virtually around the world.
Authors
Silvian Baltac, Associate Partner
Elian Pusceddu, Principal Consultant
Conor O’Sullivan, Senior Consultant
Hannah Galbraith-Olive, Consultant
Cameron Henderson, Consultant
For comments or queries, please contact the authors at: [email protected]
Disclaimer
This study was commissioned by CATF. The conclusions and recommendations do not necessarily represent the view of CATF. Whilst every effort has been made to ensure the accuracy of this report, neither CATF nor Element Energy warrant its accuracy or will, regardless of its or their negligence, assume liability for any foreseeable or unforeseeable use made of this report which liability is hereby excluded.
The Export scenario is where few storage basins are developed – CO2 emissions are aggregated at export points before being transported to a key storage basins
If only a few storage basins are developed, emitters will be reliant on aggregation at export points.
CO2 emissions will be aggregated at certain locations before being transported to a few storage basins. These export points are based on announced projects1 as well as large ports which may be likely to develop CO2 aggregation facilities due to their location.
- In 2035, it is assumed only announced projects (red) and a few key export locations are established.
- By 2050, all other locations (orange) will develop CO2 aggregation infrastructure – these export locations are driven by the location of emitters in each demand scenario. They are aggregation zones for stranded emitters and could be located elsewhere if there was demand.
Island-based export points are likely to require shipping from a small number of emitters, whereas other export points may be connected to an onshore transport network with multiple emitters connected.
The prioritised storage basins (North Sea, East Irish Sea, Aquitaine Basin, Adriatic Sea, Aegean Sea and the Black Sea) were chosen based on locations of already announced storage projects with a reasonable geographic spread in addition to well-characterised geology that has been identified as suitable for CO2 storage.
These export points are a means of aggregating national or sub-national areas of captured emissions.
- These export points are example locations; if there is significant demand from industrial emitters, it could be economic to develop an export point in closer proximity to emitters.
- In reality, there could be many more export locations developed, particularly along coastlines – this scenario is illustrative.
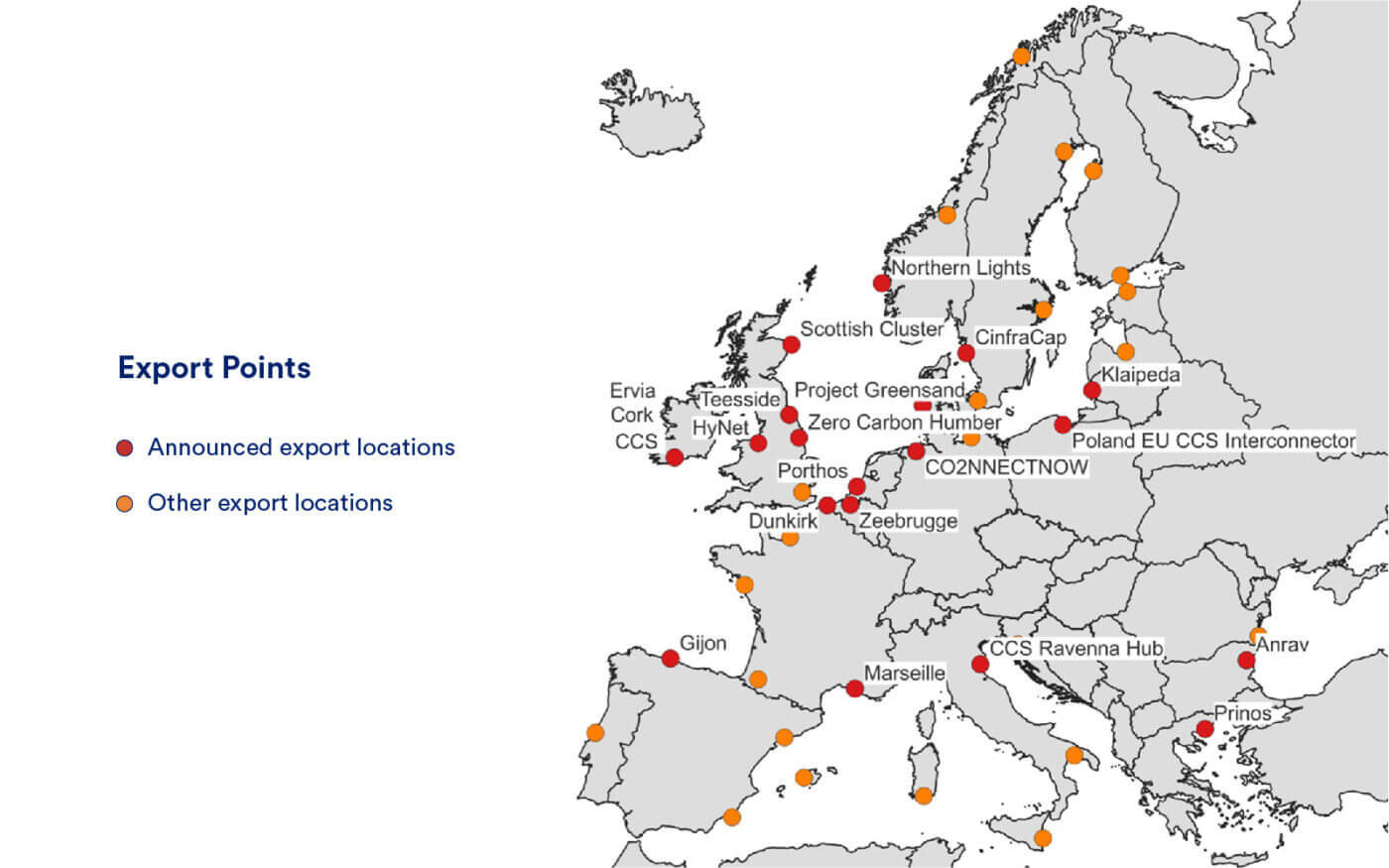
Footnotes
- Europe Carbon Capture Project Map – Clean Air Task Force (catf.us)
There is also potential to aggregate with CarbFix’s Coda Terminal but because this study is focussed on stratigraphic CO2 storage, rather than rapid mineralisation, it was not considered here.
Credits
About the Authors
This report has been prepared by Element Energy, an ERM Group company.
Element Energy is a strategic energy consultancy, specialising in the intelligent analysis of low carbon energy. The team of over 100 specialists provides consultancy services across a wide range of sectors, including the built environment, carbon capture and storage, industrial decarbonisation, smart electricity and gas networks, energy storage, renewable energy systems and low carbon transport. Element Energy provides insights on both technical and strategic issues, believing that the technical and engineering understanding of the real world challenges support the strategic work. In June 2021, Element Energy joined the ERM Group, the largest independent sustainability consultancy, with a global footprint and over 7,000 employees worldwide.
This work was commissioned by Clean Air Task Force (CATF).
Clean Air Task Force (CATF) is a global nonprofit organization working to safeguard against the worst impacts of climate change by catalysing the rapid development and deployment of low-carbon energy and other climate-protecting technologies. With 25 years of internationally recognized expertise on climate policy and a fierce commitment to exploring all potential solutions, CATF is a pragmatic, non-ideological advocacy group with the bold ideas needed to address climate change. CATF has offices in Boston, Washington D.C., and Brussels, with staff working virtually around the world.
Authors
Silvian Baltac, Associate Partner
Elian Pusceddu, Principal Consultant
Conor O’Sullivan, Senior Consultant
Hannah Galbraith-Olive, Consultant
Cameron Henderson, Consultant
For comments or queries, please contact the authors at: [email protected]
Disclaimer
This study was commissioned by CATF. The conclusions and recommendations do not necessarily represent the view of CATF. Whilst every effort has been made to ensure the accuracy of this report, neither CATF nor Element Energy warrant its accuracy or will, regardless of its or their negligence, assume liability for any foreseeable or unforeseeable use made of this report which liability is hereby excluded.
Analysis and Projections of Future CCS Demand
This study considers two scenarios for industrial CCS demand that aim to highlight the potential requirements for CO2 storage development across Europe
CCS decarbonisation pathways could be utilised by a large number of industrial facilities across Europe. The potential for carbon capture deployment across European industries was based on analysis of the Industrial Emissions Portal (IEP) database of emissions data for large point sources. This study considers two scenarios for industrial CCS demand that aim to highlight where the development of CO2 transport and storage could be required.
Technical Potential Scenario
- The Technical Potential scenario is a means of identifying the maximum possible volumes of CO2 captured. The aim of this scenario is to test the limits of geological storage capacity.
- This scenario identifies all potential sources which might require CO2 storage.
- Only the smallest scale point source emitters (<0.1 MtCO2/year) are excluded for CCS uptake in this scenario.
Prioritised CCS Scenario
- The Prioritised CCS scenario is linked to literature estimates for CCS deployment across Europe.
- This scenario represents the upper end of these literature estimates for each industrial sector.
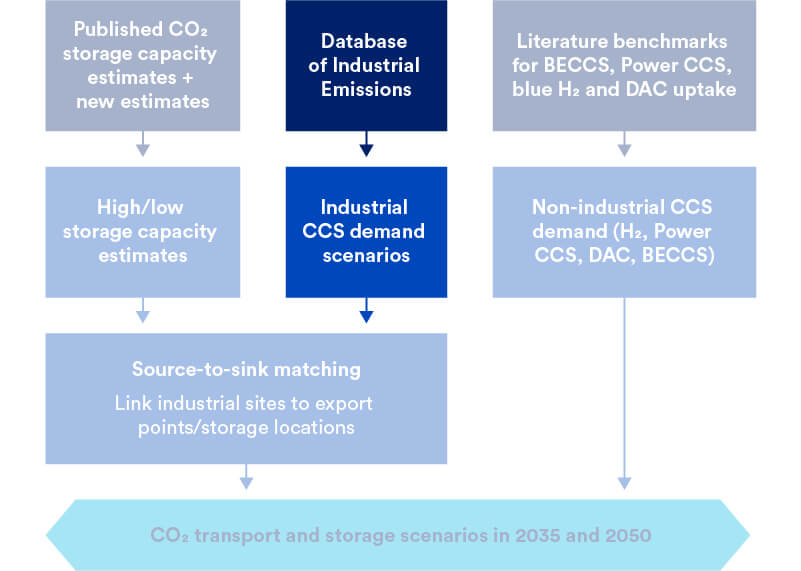
780 MtCO2/year of industrial emissions are emitted across Europe – many of which could decarbonise via CCS
Germany and Central Europe dominate total emissions reported by the Industrial Emissions Portal.
Currently the largest point source emissions are from the power generation sector (grey) which emit up to 40 MtCO2/year (both fossil and biogenic). However, there is significant uncertainty around the uptake of carbon capture within the power generation sector, so this is considered separately.
- In Europe, the largest industrial emitters include the cement & lime, chemicals, iron & steel, paper & pulp, refining and waste management sectors.
- Emissions from other sectors (white) form a minority of total emissions and are not considered for CCS uptake.
- Industrial sites are often clustered over a small geographical area for example in Rotterdam, the Rhine-Westphalia region and around Antwerp.
The aim of this study is to identify potential regions of high demand for CCS so the limits of geological CO2 storage capacity can be tested; therefore scenarios were developed to represent high CCS uptake across industrial sites.
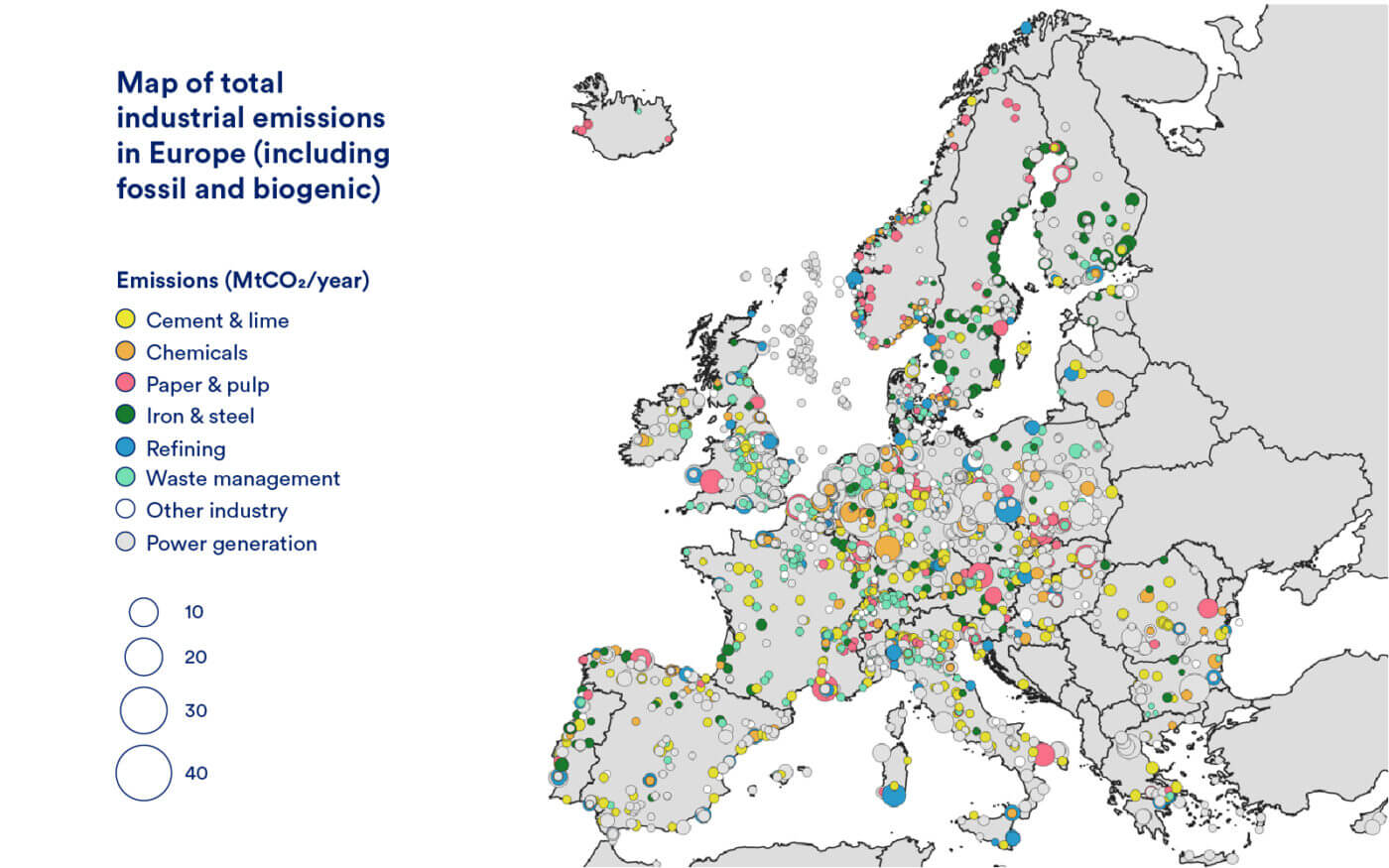
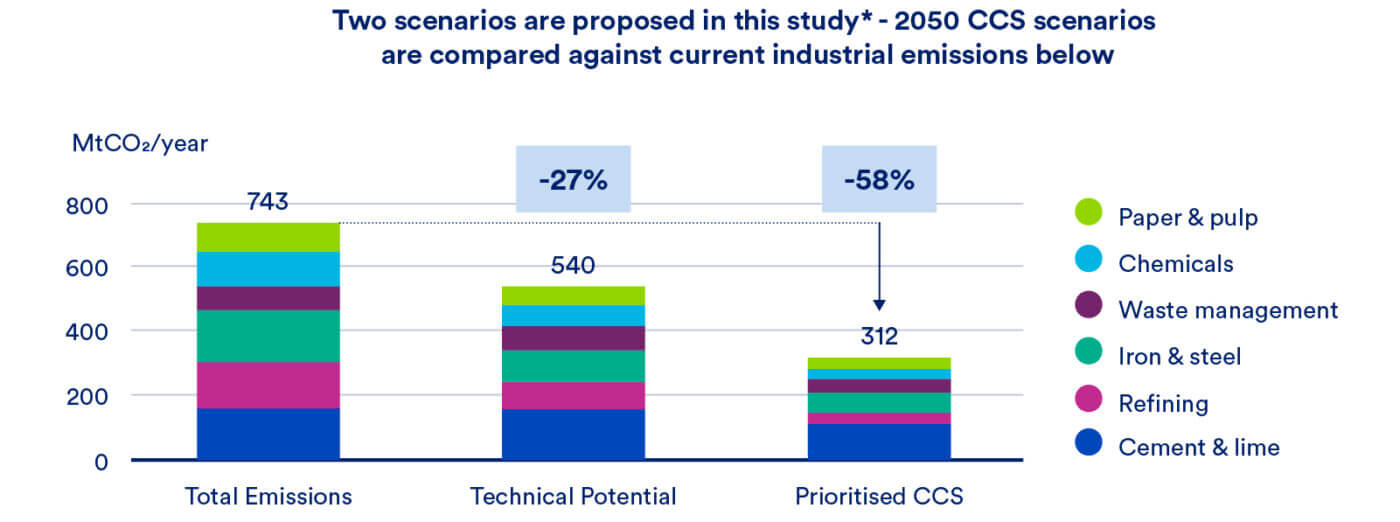
Footnotes
European Industrial Emissions Portal (europa.eu)
*The scenarios proposed in this study are not intended to represent an accurate forecast of CCS deployment in Europe. The intention is to consider how CO2 storage could be developed to meet high levels of CCS demand –identifying regions where capacity could be a constraint.
Credits
About the Authors
This report has been prepared by Element Energy, an ERM Group company.
Element Energy is a strategic energy consultancy, specialising in the intelligent analysis of low carbon energy. The team of over 100 specialists provides consultancy services across a wide range of sectors, including the built environment, carbon capture and storage, industrial decarbonisation, smart electricity and gas networks, energy storage, renewable energy systems and low carbon transport. Element Energy provides insights on both technical and strategic issues, believing that the technical and engineering understanding of the real world challenges support the strategic work. In June 2021, Element Energy joined the ERM Group, the largest independent sustainability consultancy, with a global footprint and over 7,000 employees worldwide.
This work was commissioned by Clean Air Task Force (CATF).
Clean Air Task Force (CATF) is a global nonprofit organization working to safeguard against the worst impacts of climate change by catalysing the rapid development and deployment of low-carbon energy and other climate-protecting technologies. With 25 years of internationally recognized expertise on climate policy and a fierce commitment to exploring all potential solutions, CATF is a pragmatic, non-ideological advocacy group with the bold ideas needed to address climate change. CATF has offices in Boston, Washington D.C., and Brussels, with staff working virtually around the world.
Authors
Silvian Baltac, Associate Partner
Elian Pusceddu, Principal Consultant
Conor O’Sullivan, Senior Consultant
Hannah Galbraith-Olive, Consultant
Cameron Henderson, Consultant
For comments or queries, please contact the authors at: [email protected]
Disclaimer
This study was commissioned by CATF. The conclusions and recommendations do not necessarily represent the view of CATF. Whilst every effort has been made to ensure the accuracy of this report, neither CATF nor Element Energy warrant its accuracy or will, regardless of its or their negligence, assume liability for any foreseeable or unforeseeable use made of this report which liability is hereby excluded.
This study considers two CCS demand scenarios with high levels of industrial uptake
Two scenarios illustrate the volume of CO2 that could be captured in 2035 and 2050 across major industrial sectors.
- The Prioritised CCS scenario represents the upper end of literature estimates for CCS uptake in each industrial sub-sector.
- The Technical Potential scenario aims to quantify the maximum volume of CO2 that could be captured, to test the limits of geological storage capacity and to identify sinks for all potential industrial sources which may require CCS. It results in an increase in CCS demand of over 70% compared to the Prioritised CCS scenario.
CCS uptake in each scenario was determined by percentage benchmarks in each sector.
The cement and lime sector has the highest deployment levels in both scenarios for 2035 and 2050 time periods.
CCS uptake is forecast to grow rapidly during the late 2030s and early 2040s across all sectors, with the rate of uptake plateauing towards 2050.
CCS demand is interpolated between the 2035 and 2050 values.
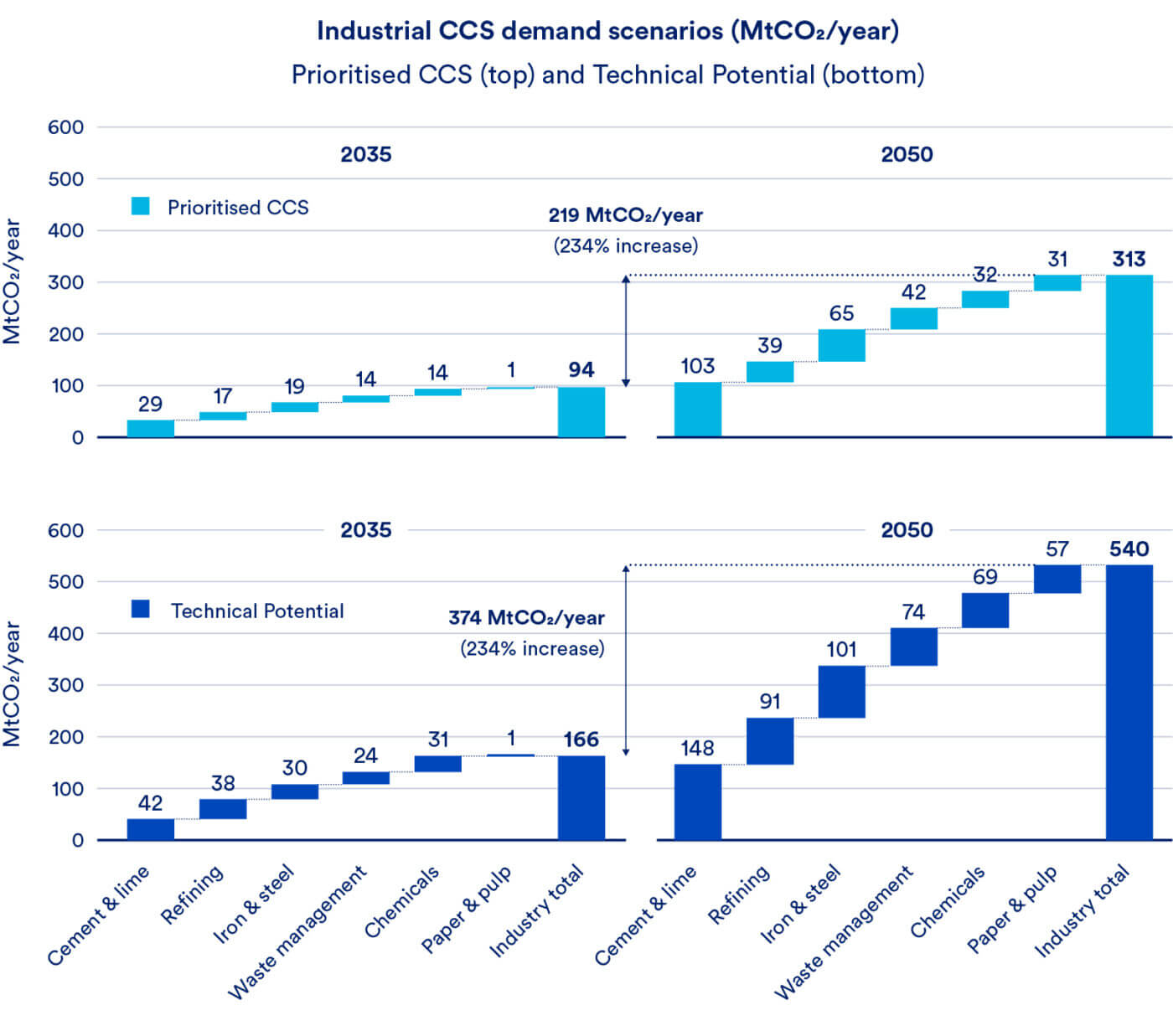
The 2035 Technical Potential scenario assumes emitters in industrial hubs or cement/waste management sectors are among the first to deploy CCS
The Technical Potential scenario in 2035 assumes emitters close to industrial hubs or in the cement/waste management sectors will be the early movers.
- By 2035, 31% of sites in the 2035 Technical Potential scenario are assumed to already be undertaking carbon capture. These sites include facilities located in industrial clusters as well as all individual sites with emissions greater than 0.5 MtCO2/year.
- Dispersed sites (i.e., away from an industrial hub) are assumed to have not yet installed carbon capture facilities unless they are part of the cement and waste management sectors which are likely to be early movers in carbon capture installations.
- Capture deployment in 2035 is assumed to be below 100% at the facility level. This represents the phased deployment of capture (e.g., where CCS will be applied to multiple emitting streams) as well as ramp-up limitations of CO2 transport and storage infrastructure.
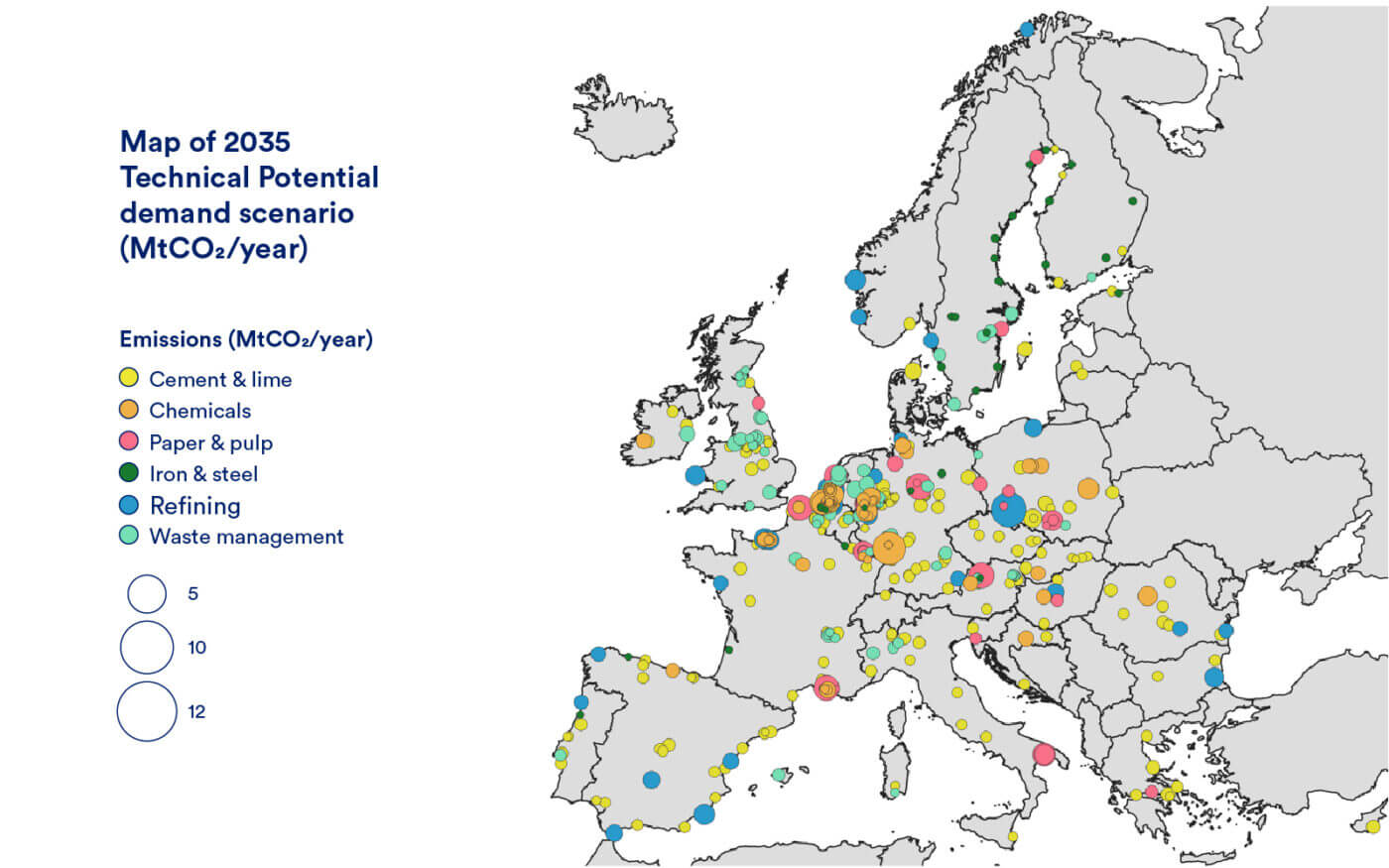
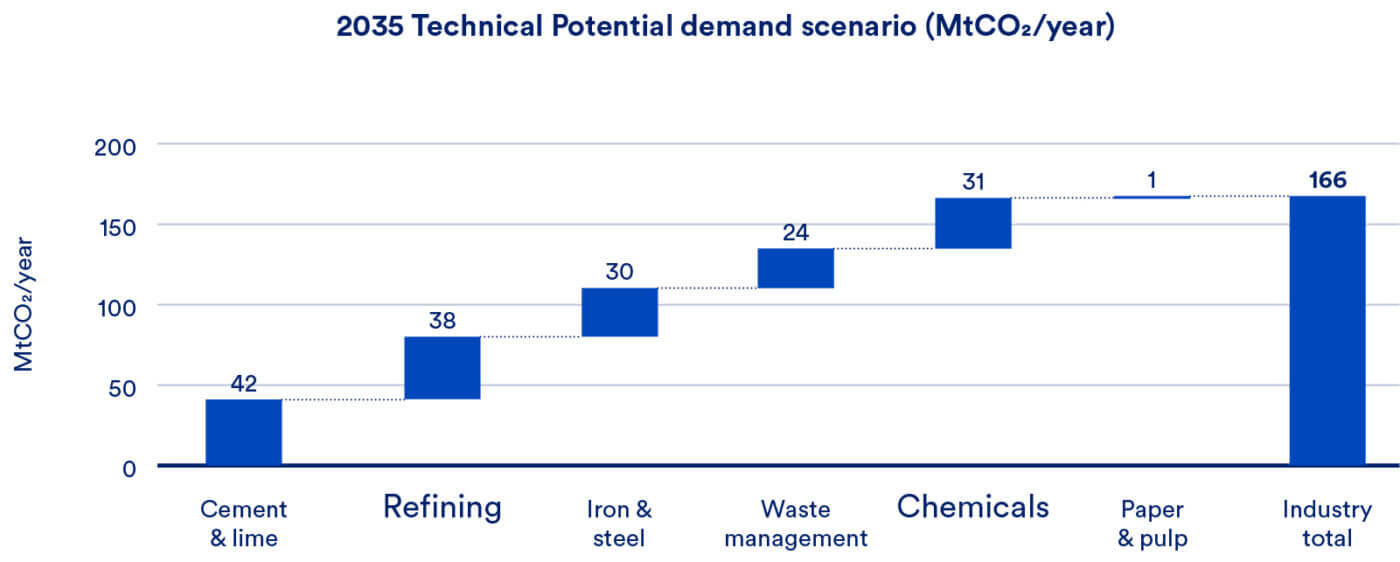
The 2050 Technical Potential scenario depicts the use of CCS at every industrial site across Europe
The Technical Potential scenario in 2050 gives 520 MtCO2/year as the maximum amount of industrial carbon capture across Europe.
- All current industrial sites with capturable emissions >0.1 MtCO2/year are included in the Technical Potential scenario. No prediction is made for possible de-industrialisation in Europe.
- For each sector, a fraction of present-day emissions is assumed to be captured based on their industrial processes. Capture rates are dependent on the emitting streams of each sub-sector – with high purity and the largest emitting streams representing the most economically viable options for high levels of capture.
- Capture benchmarks are higher in the Technical Potential scenario for all sectors, resulting in a greater overall volume of CO2 emissions captured.
- The 2050 Technical Potential scenario has 520 MtCO2/year being captured across 1380 industrial sites.
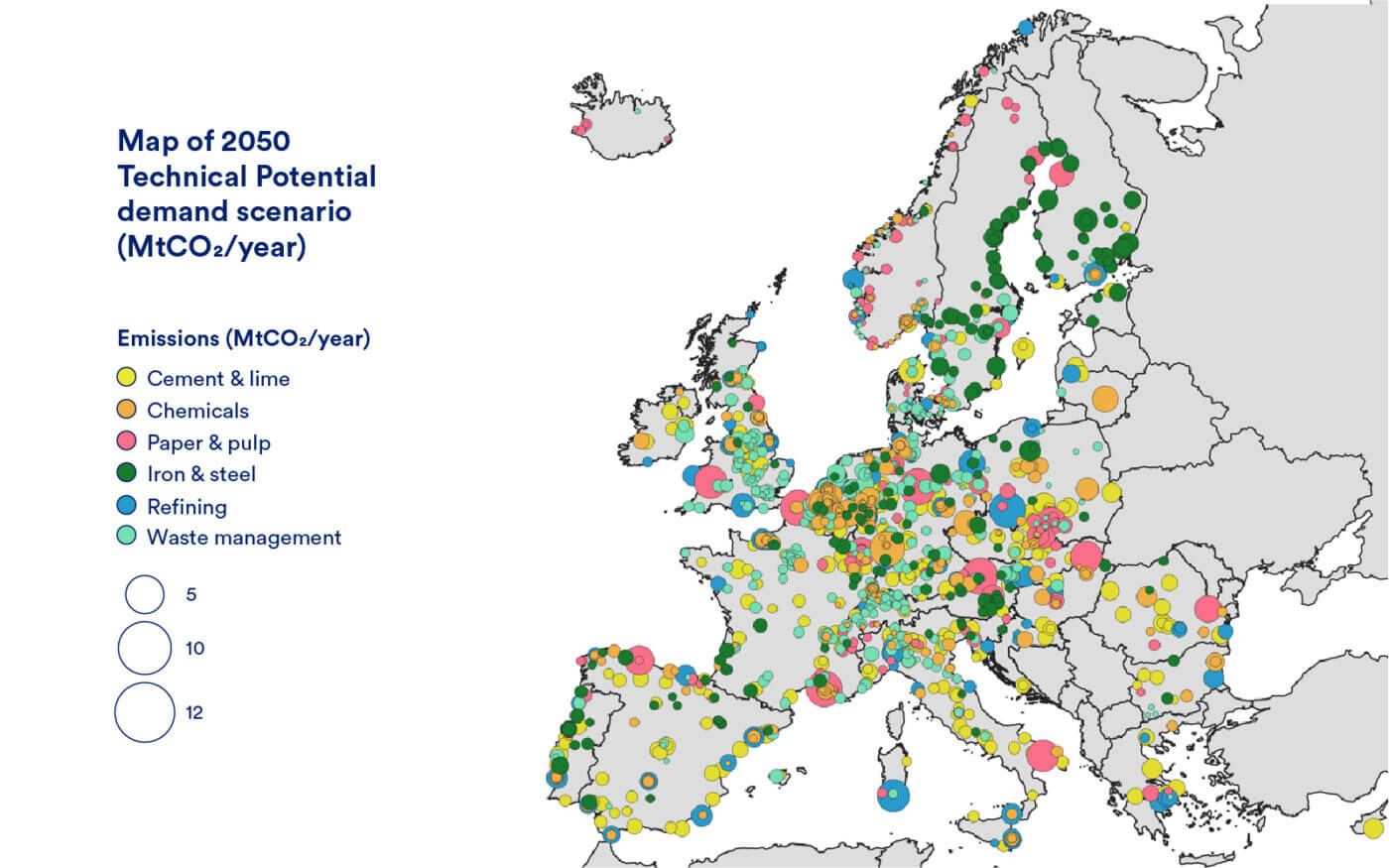
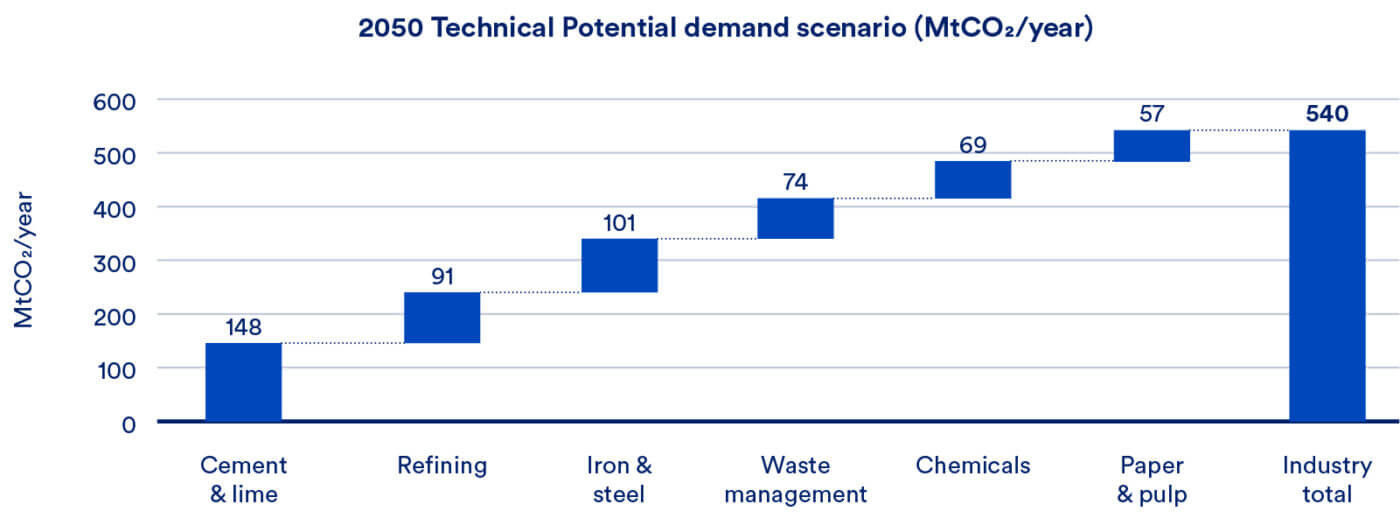
In the 2035 Prioritised CCS scenario, large-scale emitters and the cement sector are most likely to install a carbon capture facility first
The Prioritised CCS scenario in 2035 illustrates limited uptake of carbon capture in sectors other than cement.
- In 2035, large scale emitters in major industrial hubs (>7 MtCO2) are prioritised for CCS uptake. These sites are located within industrial clusters as well as large-scale cement and lime facilities.
- However, the cement sector is likely to deploy CCS even in smaller-scale dispersed sites due to the lack of alternative decarbonisation options.
- Capture deployment in 2035 is assumed to be below 100% at the facility level. This represents the phased deployment of capture (e.g., where CCS will be applied to multiple emitting streams) as well as ramp-up limitations of CO2 transport and storage infrastructure.
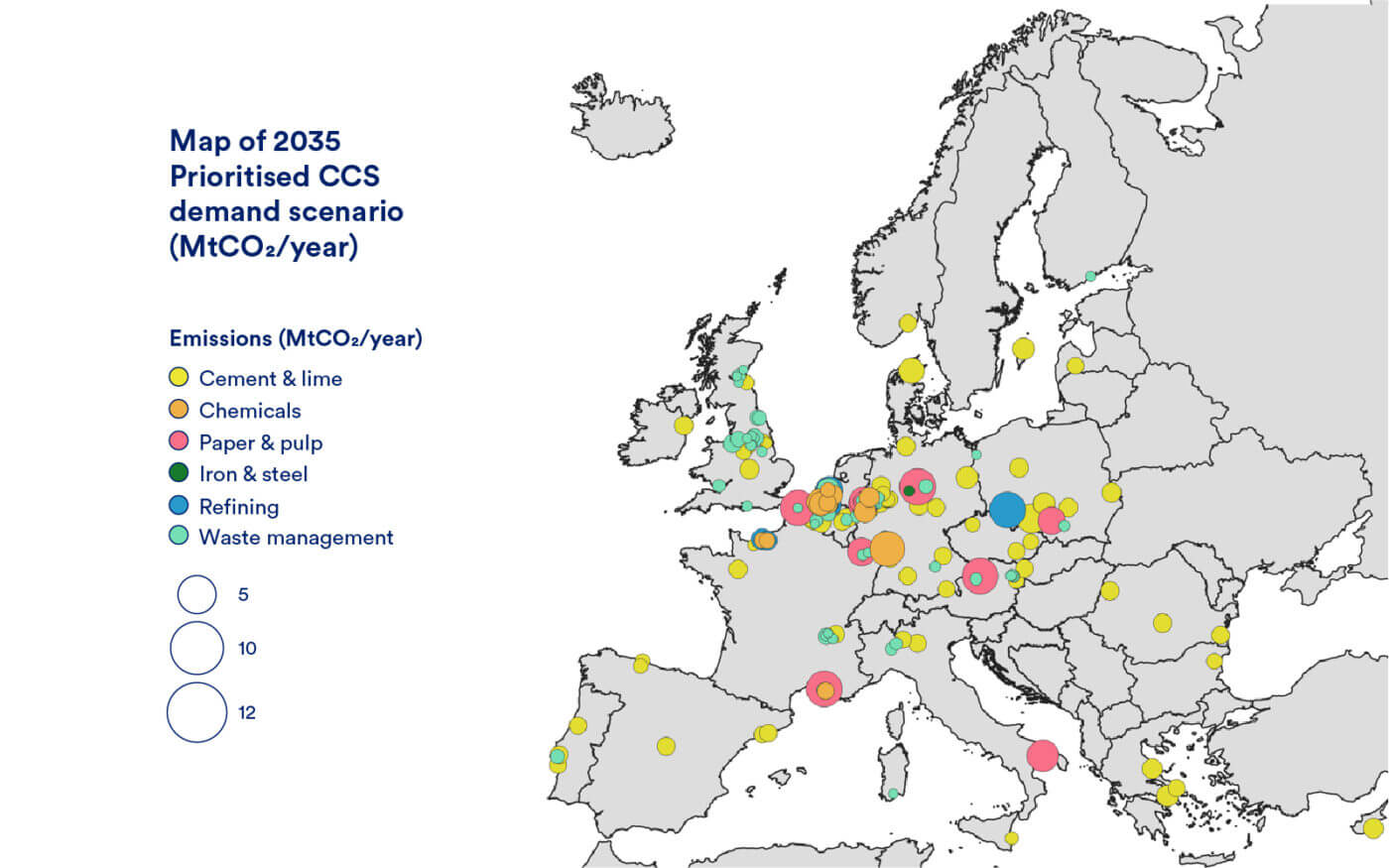
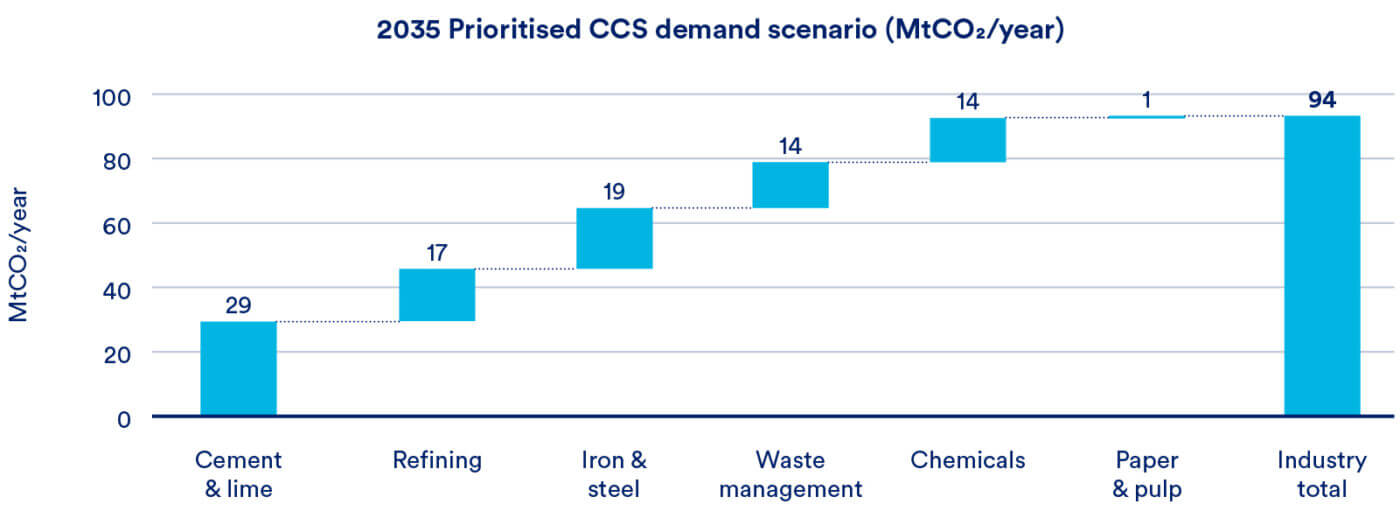
Emitters are scored based on how likely they are to deploy CCS, the highest scoring emitters are included in the 2050 Prioritised CCS scenario
The Prioritised CCS scenario is proposed as a reasonable outcome if CCS is one of the favoured decarbonisation option in Europe.
A scoring system is used to prioritise which industrial sites are most likely to deploy CCS in the Prioritised CCS scenario. This considers:
- Sub-sector dependence on CCS (i.e., availability of alternative decarbonisation pathways)
- BECCS and negative emissions potential
- Scale of facility emissions – economies of scale mean large sites are more likely to install a carbon capture facility
- Proximity to an industrial cluster/hub
Sites with the highest scores are included in the Prioritised CCS scenario, with the volume of captured emissions a certain fraction of present-day emissions dependent on sub-sector.
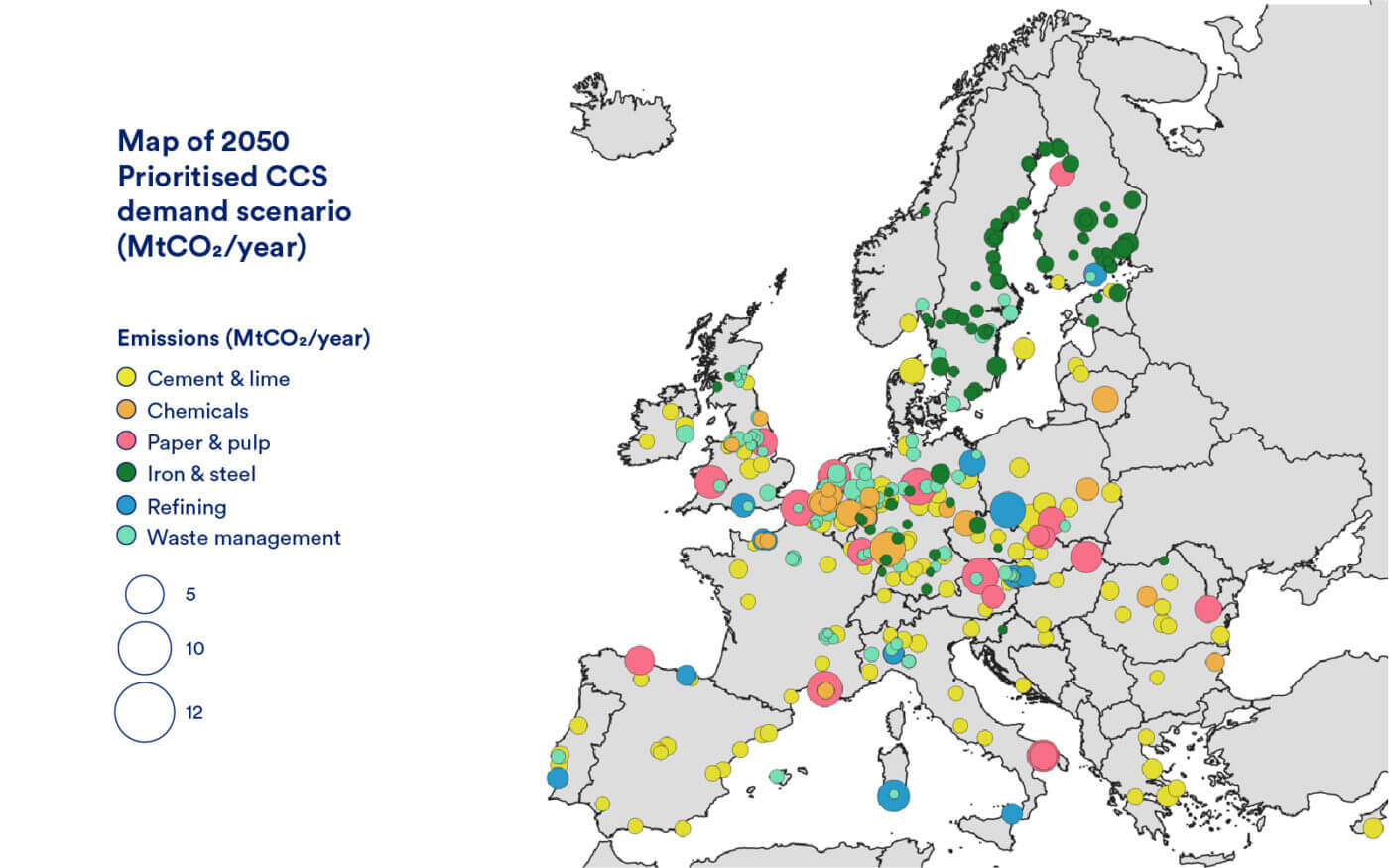
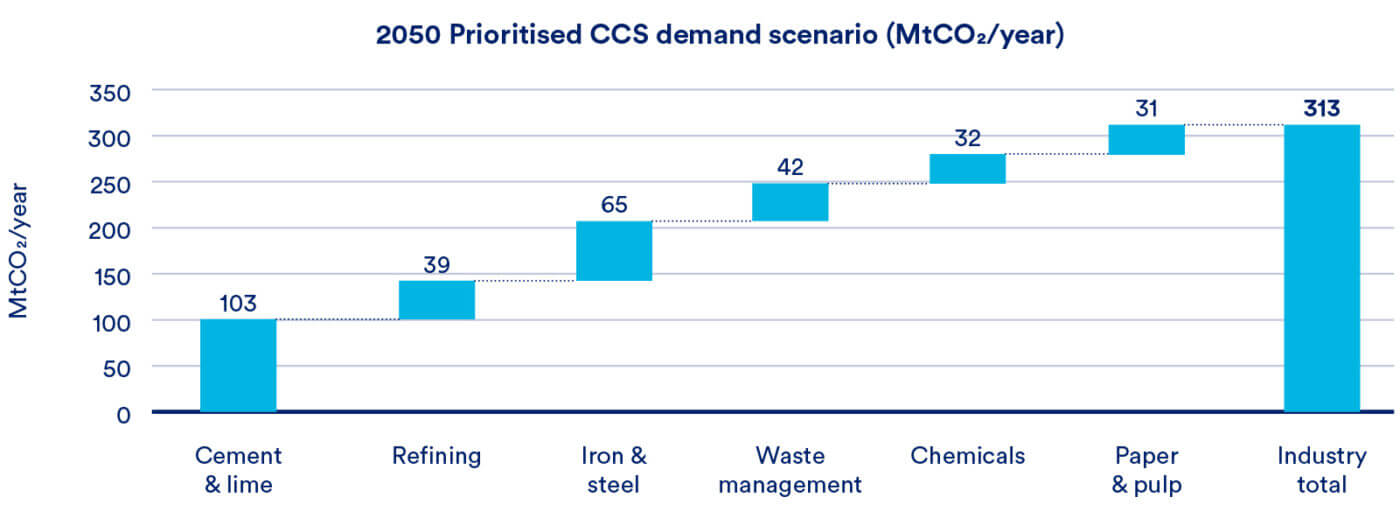
Non-industrial CCS demand is highly uncertain but could be a significant driver for CO2 storage development in the future
Scenario development for non-industrial CO2 demand:
CCS demand from non-industrial sectors, although highly uncertain, is likely to represent significant volumes of emissions capture. This study considers CCS demand from the four sectors expected to account for the majority of non-industrial emissions. Blue hydrogen, power CCS, bioenergy CCS (BECCS) and direct air capture (DAC) technologies are likely to play an essential role in ensuring Europe achieves its climate goals.
Blue Hydrogen
- Many blue hydrogen projects have already been announced across Europe, with several countries planning on developing significant production capacity as part of their future decarbonisation plaans.
- Blue hydrogen could replace significant volumes of existing grey hydrogen demand in industry, whilst also supplying a growing demand for hydrogen in the transport, heat and power sectors.
Power CCS
- CCS capacity in the power sector could increase significantly by 2050 to provide dispatchable power at times when renewable generation is low –particularly if the development of energy storage capacity is limited.
BECCS & DAC
- High deployment of CO2 removals is likely to be required to offset residual emissions from hard-to-abate sectors.
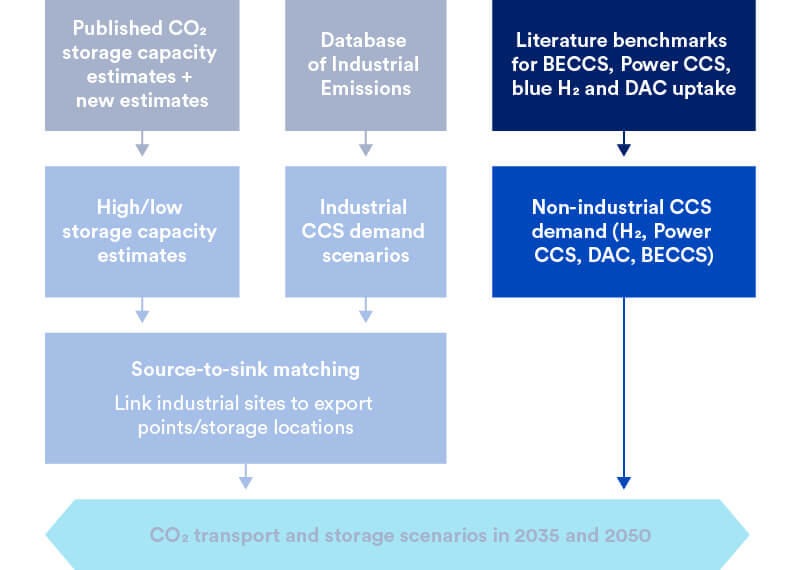
Non-industrial CCS demand contributes up to an additional 720 MtCO2/year by 2050
European level allocation
- Data required to determine the location of non-industrial emissions was beyond the scope of this study.
- Key drivers required to determine the location of future DAC and blue hydrogen capacity are unknown or highly uncertain, therefore demand is considered at a European level.
- DAC and blue hydrogen development is likely to be driven by the availability of geological storage capacity.
- Countries with surplus storage capacity (once industrial emissions have been stored) are identified in source-to-sink matching.
Country level allocation
- Country level allocation is considered for power CCS based on existing fossil-based capacity of power generation assets for each country.
- Country level allocation is considered for BECCS capacity based primarily on industrial sectors with the highest potential for negative emissions (paper & pulp, waste management).
- Existing biogenic emissions from power are considered as a secondary factor.
Country level allocation for power CCS and BECCS provides an overview of how non-industrial demand could be distributed geographically in Europe. However, it should be noted that this analysis does not consider many of the drivers that would influence uptake in these sectors.
Non-industrial CCS demand contributes up to an additional 720 MtCO2/year by 2050
Benchmarks for non-industrial CCS demand in Europe
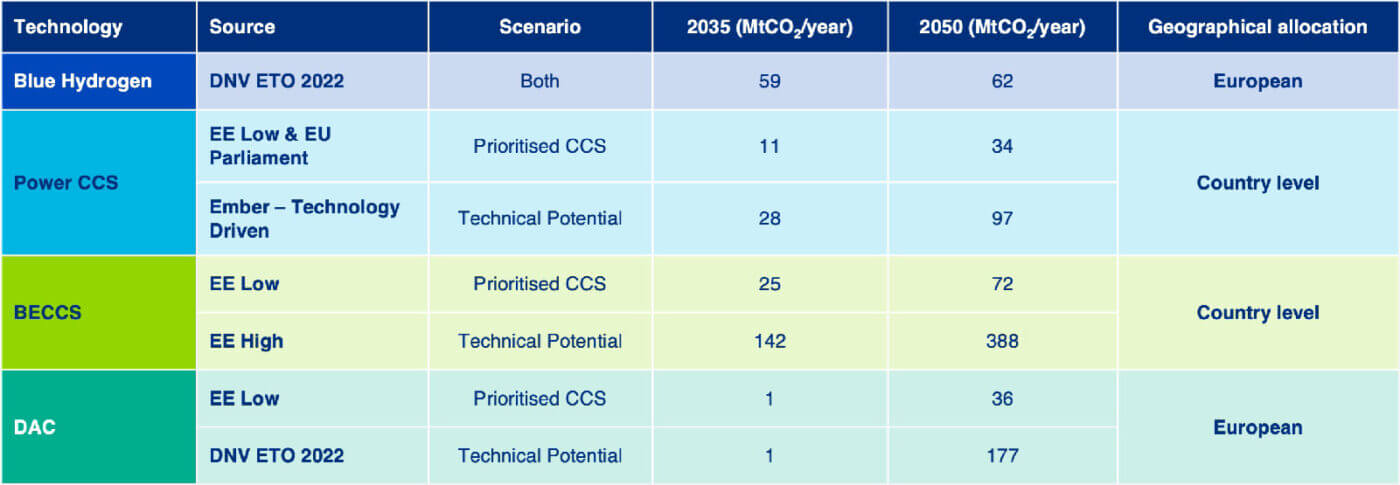
Demand for industrial CCS is dominant, when compared to non-industrial demand, for all countries in the Prioritised CCS scenario
CCS demand from BECCS and Power CCS is equivalent to an 11% median increase from country level industrial demand in 2035
- Sweden and Finland are the key outliers due to their large paper and pulp sectors representing significant potential for negative emissions from BECCS.
- Given the current large power sector in Germany, it represents a significant potential for Power CCS in both 2035 and 2050.
Blue hydrogen and DAC demand is considered separately due to uncertainties in deployment locations
- Demand from blue hydrogen and DAC across Europe exceeds the industrial demand of Germany in 2035.
- By 2050 in the Prioritised CCS scenario, non-industrial demand accounts for an additional demand of 204 MtCO2/year – this is an additional 65% demand compared to industrial sources in the 2050 Prioritised CCS scenario.
- The location of blue hydrogen and DAC developments are likely to be driven by availability of geological storage capacity and access to CO2 T&S infrastructure.
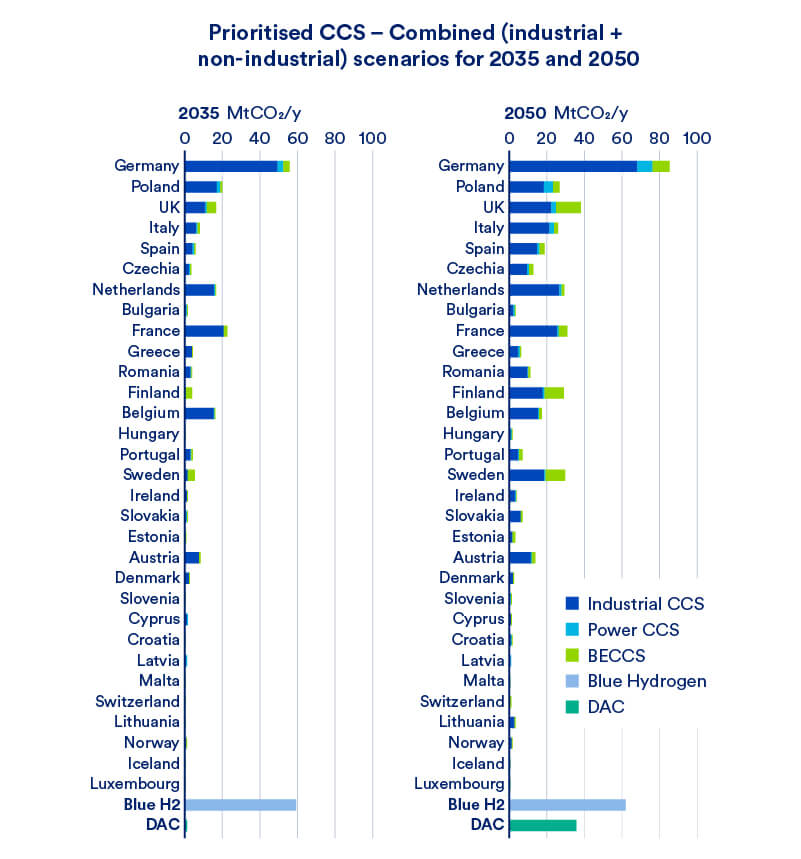
Industrial CCS is also dominant for the majority of countries in the Technical Potential scenario, but there is significant BECCS demand from Finland, Sweden and the UK
Industrial demand is significantly greater than that from power CCS and BECCS for most countries in the Technical Potential scenario
- Median industrial CCS demand as a percentage of country-allocated CCS is 71% in 2035 and 63% in 2050.
- Power CCS demand is relatively small for all countries with a median allocated demand of 6% in 2035 and 8% in 2050.
- BECCS demand is greater than industry for the UK, Finland, Sweden and Estonia in both 2035 and 2050.
CCS demand from blue hydrogen and DAC could be a significant driver in the development of transport and storage infrastructure across Europe
- By 2050 in the Technical Potential scenario across Europe, DAC alone could add an extra 33% to the demand for CCS.
- In total, non-industrial demand in the 2050 Technical Potential scenario is 724 MtCO2/year, or 1.3 times higher than industrial demand in the 2050 Technical Potential scenario.
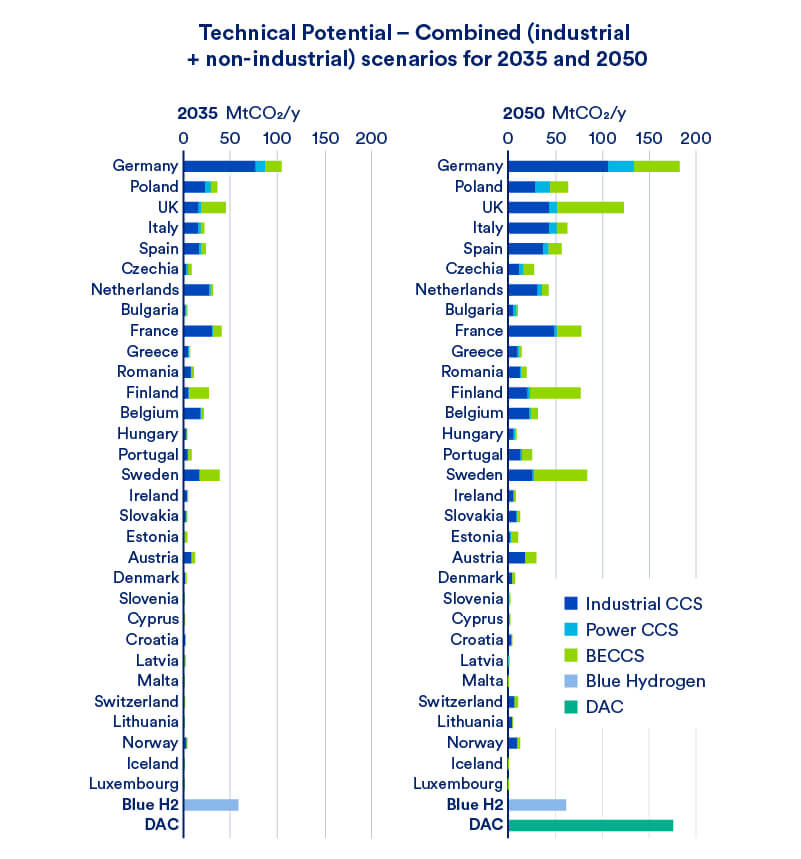
Source-sink Matching Scenarios
“Years of theoretical storage capacity” is used as a key metric to compare low/high storage capacity estimates and the amount of carbon captured in each industrial scenario
In this section, scenarios are developed in 2035 and 2050 for the level of CCS required in each country.
- By comparing countries with high CO2 demand and countries with high CO2 storage capacity, locations are identified where investment in CCS infrastructure will be most impactful.
- These results can be used to identify, for example, regions of high emissions in close proximity to developing CO2 storage sites, which could be promising for investment in CO2 transport and storage.
Comparison between geological storage capacity and demand scenario is made using “years of theoretical storage capacity.”
To compare these storage capacities with each industrial scenario, a key metric for comparison is “years of storage capacity”.
- This assumes CO2 is stored at a constant rate, equal to 100% of emissions captured – in reality, storage rates are likely to be smaller in the short term and work up to a maximum value.
- Therefore, this metric represents the fewest years theoretical storage can be utilised.
“Years of theoretical storage capacity” highlights locations that are likely to have a constraint on storage capacity.
However, estimates made in this study and some literature estimates refer to the theoretical capacity whereas other estimates from the literature are based on the effective capacity (i.e., account for traps within the prospective storage reservoir).
In practice, only a fraction of this theoretical/effective CO2 storage capacity can actually be utilised for a variety of technical, economic, legal and social reasons.
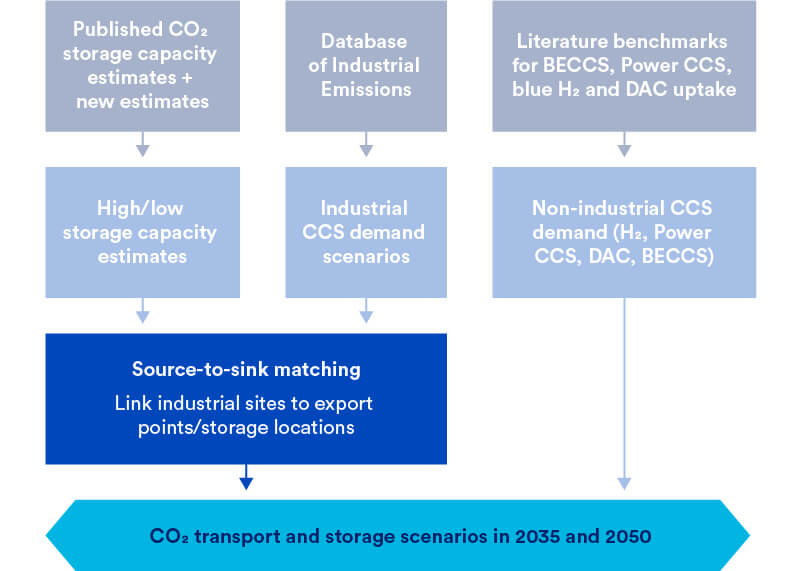
Considering industrial emitters in the 2050 Technical Potential scenario, there is storage availability for 500-2900 years across Europe
- Across Europe there is 500-2900 years of theoretical storage capacity available for the level of demand of the Technical Potential scenario in 2050, where CCS demand from industry reaches 520 MtCO2/year.
- Norway has enough capacity to support CCS in the Technical Potential scenario for up to 36,000 years of its own emissions whereas Austria only has a maximum of 29 years capacity in the Technical Potential scenario. This assumes CCS demand is constant across all years.
- 19 countries (68%) have enough CO2 storage capacity to store their own emissions for over 100 years and 20 countries have enough to store their own emissions for over 50 years under the Low estimate.
- For the High estimate, 23 countries (82%) have CO2 storage capacity to store their own emissions for over 100 years.
- Austria, Belgium, Estonia, Finland and Luxembourg are all likely to have to export their captured emissions to another country.
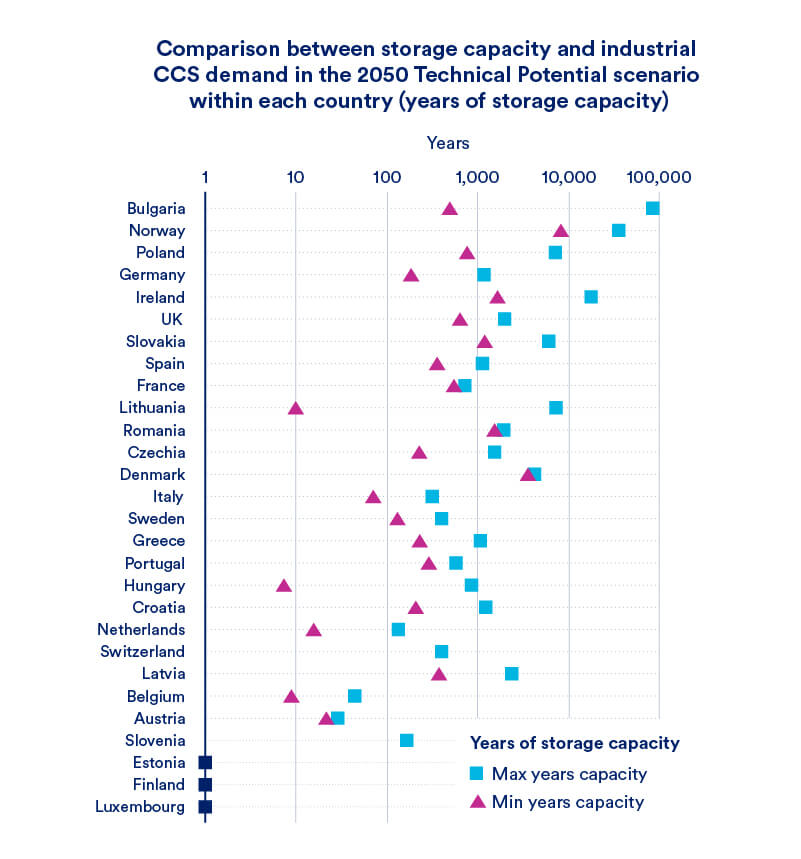
2050 Prioritised CCS industrial demand is 313 MtCO2/year – across Europe there is 820 years of storage capacity in the Low scenario and >4700 years in the High scenario
- Norway has enough capacity to support CCS in the Prioritised CCS scenario for up to 175,000 years of its emissions whereas Germany has a minimum of 290 years capacity in the Prioritised CCS scenario. This assumes CCS demand is constant across all years.
- 20 countries (71%) have enough CO2 storage capacity to store their own emissions for over 100 years under the Low estimate.
- For the High estimate, 23 countries (82%) have CO2 storage capacity to store their own emissions for over 100 years.
- Belgium, Netherlands, Estonia, Finland and Luxembourg are all likely to have to export their captured emissions to another country.
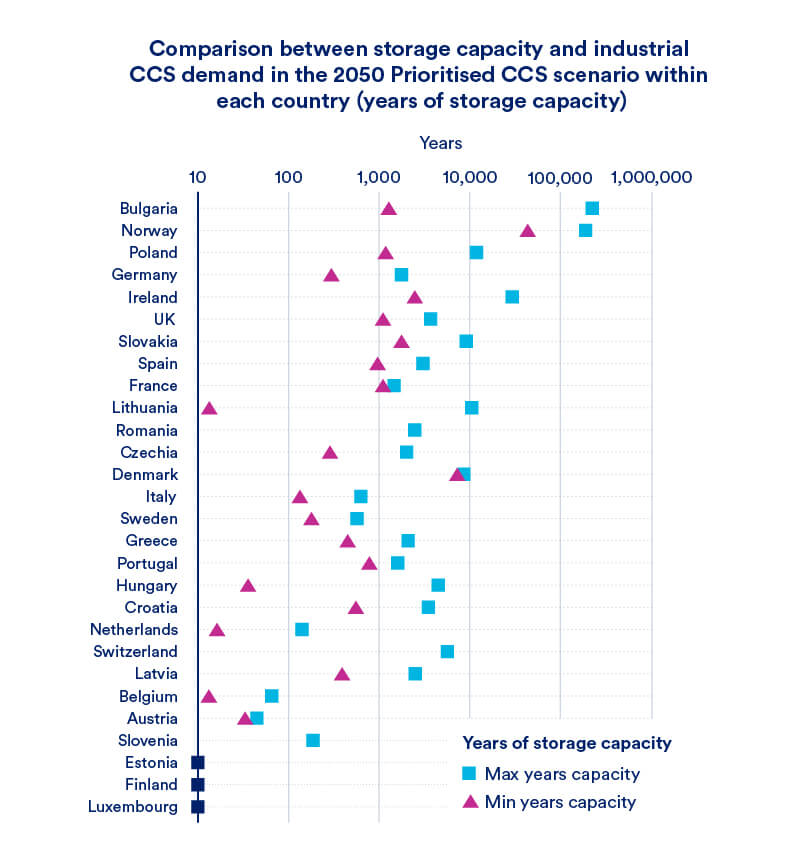
Even with the addition of non-industrial demand there is still at least 200 years of theoretical storage capacity within Europe considering the highest demand scenarios in 2050
- In the low storage capacity scenario, Lithuania, Hungary, the Netherlands and Belgium have ≤10 years of storage.
- Belgium, Austria and Slovenia also have ≤100 years of storage in the high storage capacity scenario.
- Germany, Italy and Sweden may also be at risk of reaching their storage capacity limits if Power CCS and BECCS are also considered.
- If all non-industrial demand (i.e., DAC, Blue H2, Power CCS and BECCS) is considered, along with the 2050 Technical Potential scenario, demand would be 1250 MtCO2/year, across Europe.
- This would give at least 200 years storage capacity in the low scenario or 1213 years in the high storage capacity scenario.
- There is likely to be available capacity in Bulgaria, Norway, Ireland and the UK – these are likely to be the countries which develop DAC and Blue H2 facilities due to their close proximity to favourable storage locations.
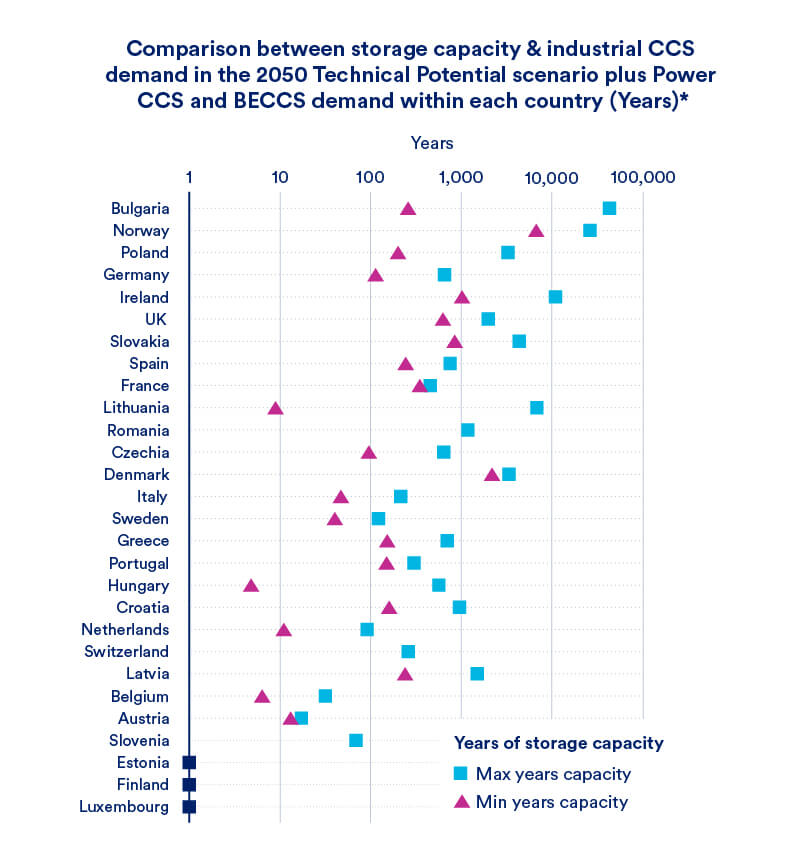
However, if storage capacity is limited to announced project capacities –when compared to 2035 Prioritised CCS demand, there is a minimum of 27 years storage capacity
- If all non-industrial demand (i.e., DAC, Blue H2, Power CCS and BECCS) in 2035 is considered, along with the 2035 Prioritised CCS scenario, demand would be 190 MtCO2/year.
- If this demand is compared to announced storage project capacity (5100 MtCO2), it would give just 27 years of actual storage capacity.
- If CCS were to become a widespread decarbonisation option, far more storage capacity needs to be developed than what is already planned.
- It currently takes ~3-4 years to develop an offshore storage reservoir – CO2 storage development is likely to be a limiting factor for short-term CCS deployment.
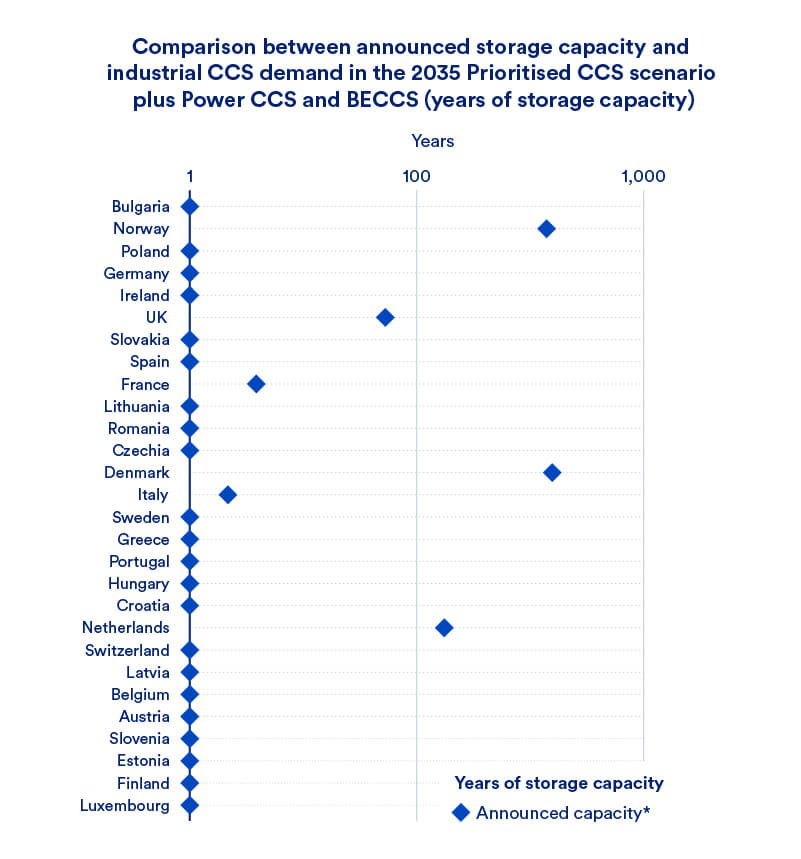
The source-to-sink scenarios aim to illustrate a wide variety of possible transport and storage solutions for industrial CCS across Europe
By considering the location of industrial emitters and geological storage capacity, 8 source-to-sink scenarios were developed.
Source-to-sink matching for 4 configurations (1-4) of CCS demand (Technical Potential vs Prioritised CCS) and CO2 storage availability (Domestic vs Export) were considered. Each of these scenarios is analysed in both 2035 (a) as well as 2050 (b) to give 8 source-to-sink scenarios (1a-4b) in total.
These scenarios highlight where support of CCS development is likely to be most impactful, such as regions of high emissions which could benefit most from accelerated investment in CO2 transport and storage infrastructure.
Limitations of this analysis
- The location of storage sites/export points used in this analysis may not necessarily be where this infrastructure is developed.
- Feasibility of transport routes was not considered – lines joining emitters and storage sites/export points do not represent actual transport routes.
- No limits have been set for maximum transport distance between storage projects/export points.
- Non-industrial demand is not considered in this source-to-sink matching due to high levels of uncertainty associated with the location of future deployment.
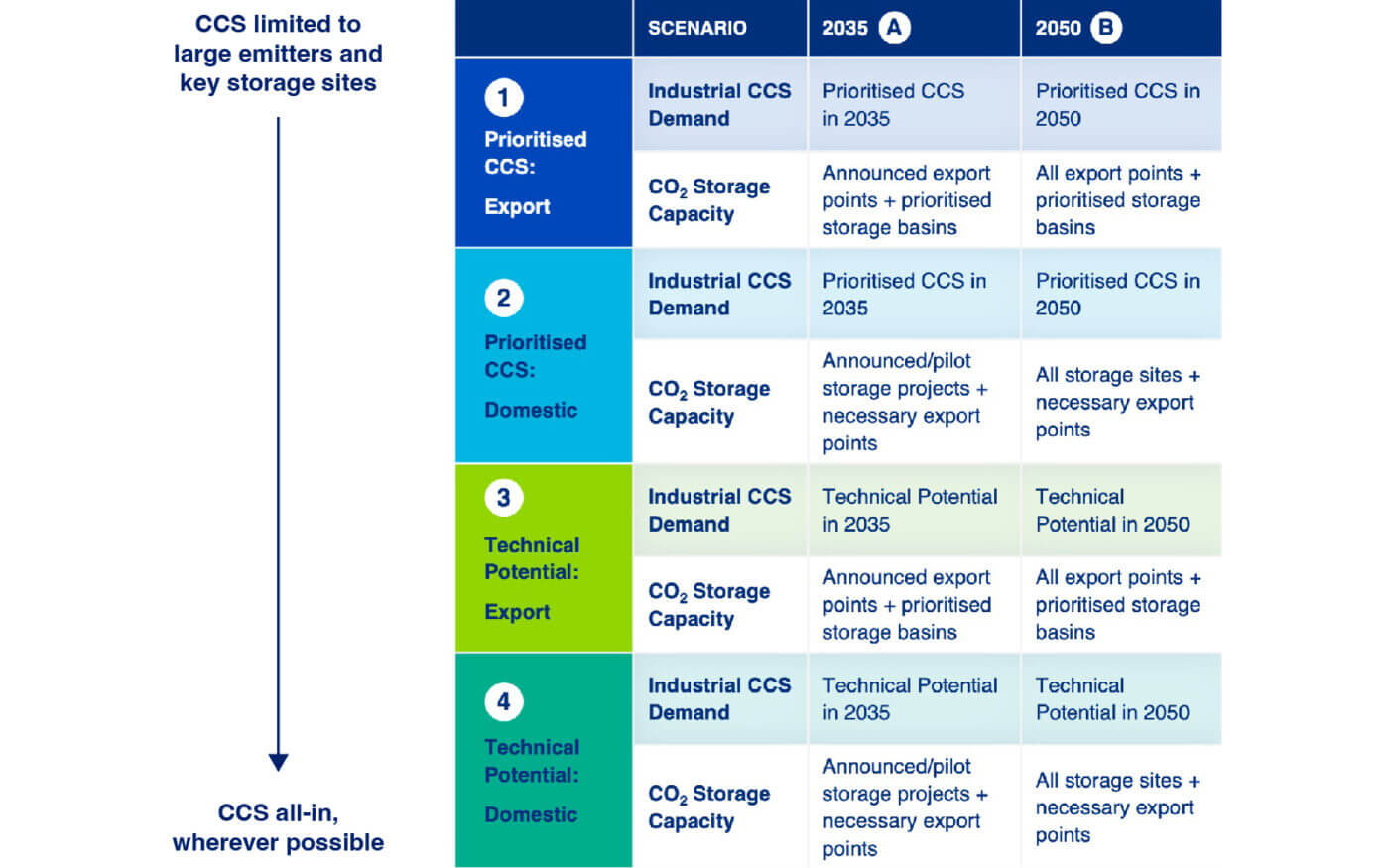
For CCS to be viable for inland emitters in the 2035 Prioritised CCS / Export scenario, onshore transport networks need to be established
In the 2035 export scenario, only announced export locations are developed along with a few key export locations in the Mediterranean.
- Export points around the North and Baltic Seas are crucial; connecting inland emitters to these will encourage widespread CCS adoption.
- Export locations need to be developed in the Mediterranean if CCS is to become a viable decarbonisation option.
Most emissions are stored in the North Sea, but there should be enough theoretical storage capacity in Northern Italy for at least 100 years in this scenario.
- In this scenario, 80% of emissions (76 MtCO2/year) are allocated via export locations to the North Sea, which gives 1300-4700 years of theoretical storage at 2035 capture rates.
- 23 MtCO2/year emissions are aggregated at an export point in Northern Germany –building an onshore pipeline network will enable decarbonisation via CCS for large emitters in NW Germany.
- However, 14 MtCO2/year of emissions are allocated to storage sites in the Adriatic Sea. This could give around 100-450 years’ worth of theoretical storage capacity in the wider Northern Italy region*.
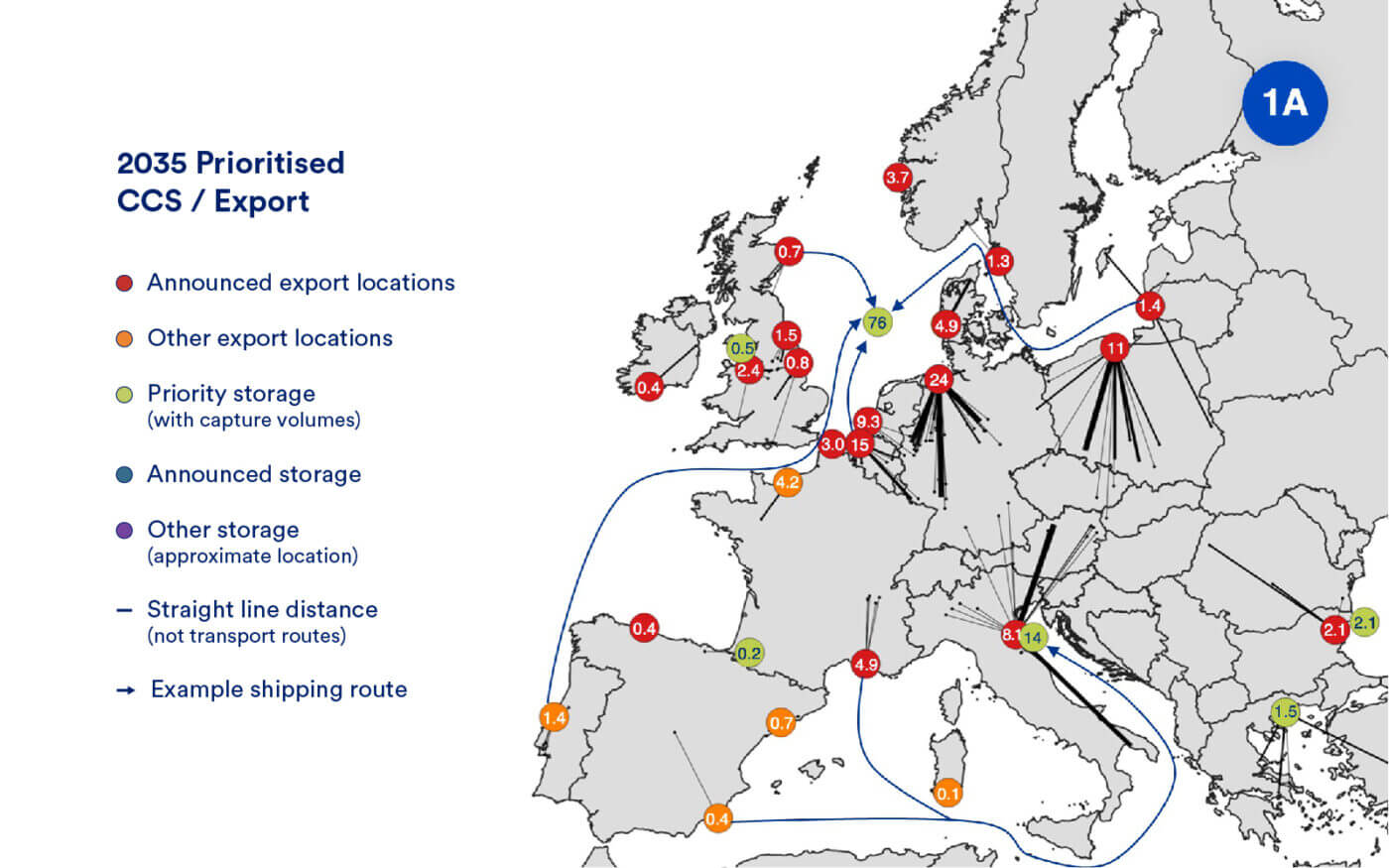
For CCS to be viable for inland emitters in the 2035 Prioritised CCS / Export scenario, onshore transport networks need to be established
Onshore transport costs will be incurred from the emitter to the export location and additional costs will arise from offshore transport to the priority storage site.
- Onshore transportation will cost €2.5-4.6/tCO2 for pipeline transport, depending on CO2 flow rates1.
- If offshore pipelines were used to transport the emissions from export points and storage sites, this could add between €6.5-30.4/tCO2 whereas using shipping would add €9.6-16.9/tCO21.
- Therefore, the overall CAPEX cost up to 2035 for the Prioritised CCS / Export scenario is €3.6-12.5 billion and the OPEX for 2035 is €0.6-1.7 billion/year.
Footnotes
- Element Energy – CO2 Shipping Model for BEIS for transport along 100 km long onshore pipelines at a rate of 0.5-2 MtCO2/year, 200 km offshore pipelines between 0.6-10 MtCO2/year and/or 500 km via ships at a rate of 0.5-9 MtCO2/year.
Credits
About the Authors
This report has been prepared by Element Energy, an ERM Group company.
Element Energy is a strategic energy consultancy, specialising in the intelligent analysis of low carbon energy. The team of over 100 specialists provides consultancy services across a wide range of sectors, including the built environment, carbon capture and storage, industrial decarbonisation, smart electricity and gas networks, energy storage, renewable energy systems and low carbon transport. Element Energy provides insights on both technical and strategic issues, believing that the technical and engineering understanding of the real world challenges support the strategic work. In June 2021, Element Energy joined the ERM Group, the largest independent sustainability consultancy, with a global footprint and over 7,000 employees worldwide.
This work was commissioned by Clean Air Task Force (CATF).
Clean Air Task Force (CATF) is a global nonprofit organization working to safeguard against the worst impacts of climate change by catalysing the rapid development and deployment of low-carbon energy and other climate-protecting technologies. With 25 years of internationally recognized expertise on climate policy and a fierce commitment to exploring all potential solutions, CATF is a pragmatic, non-ideological advocacy group with the bold ideas needed to address climate change. CATF has offices in Boston, Washington D.C., and Brussels, with staff working virtually around the world.
Authors
Silvian Baltac, Associate Partner
Elian Pusceddu, Principal Consultant
Conor O’Sullivan, Senior Consultant
Hannah Galbraith-Olive, Consultant
Cameron Henderson, Consultant
For comments or queries, please contact the authors at: [email protected]
Disclaimer
This study was commissioned by CATF. The conclusions and recommendations do not necessarily represent the view of CATF. Whilst every effort has been made to ensure the accuracy of this report, neither CATF nor Element Energy warrant its accuracy or will, regardless of its or their negligence, assume liability for any foreseeable or unforeseeable use made of this report which liability is hereby excluded.
In the 2050 Prioritised CCS / Export scenario additional onshore transport networks need to be developed in Poland, Italy and Croatia by 2050
By 2050, more export points have been developed to serve the increasing number of emitters using CCS as a decarbonisation option.
- Many coastal emitters are likely to develop their own export location.
- Storage is still limited to a few key basins.
A smaller proportion of emissions are allocated to the North Sea, but capacity could be limited in Northern Italy.
- By 2050, a smaller fraction of emissions (72% of emissions, 228 MtCO2/year) are allocated via export locations to the North Sea. This gives 430-1600 years of theoretical storage.
- Furthermore, 21% of emissions (64 MtCO2/year) are allocated to storage sites in the Adriatic Sea – resulting in just 23-100 years’ worth of theoretical capacity in Northern Italy.*
- Given the potential technical limitations of geological CO2 storage capacities, other storage sites in the Mediterranean should be used instead – such as in the Aegean or Black Seas which both have sufficient storage capacities.
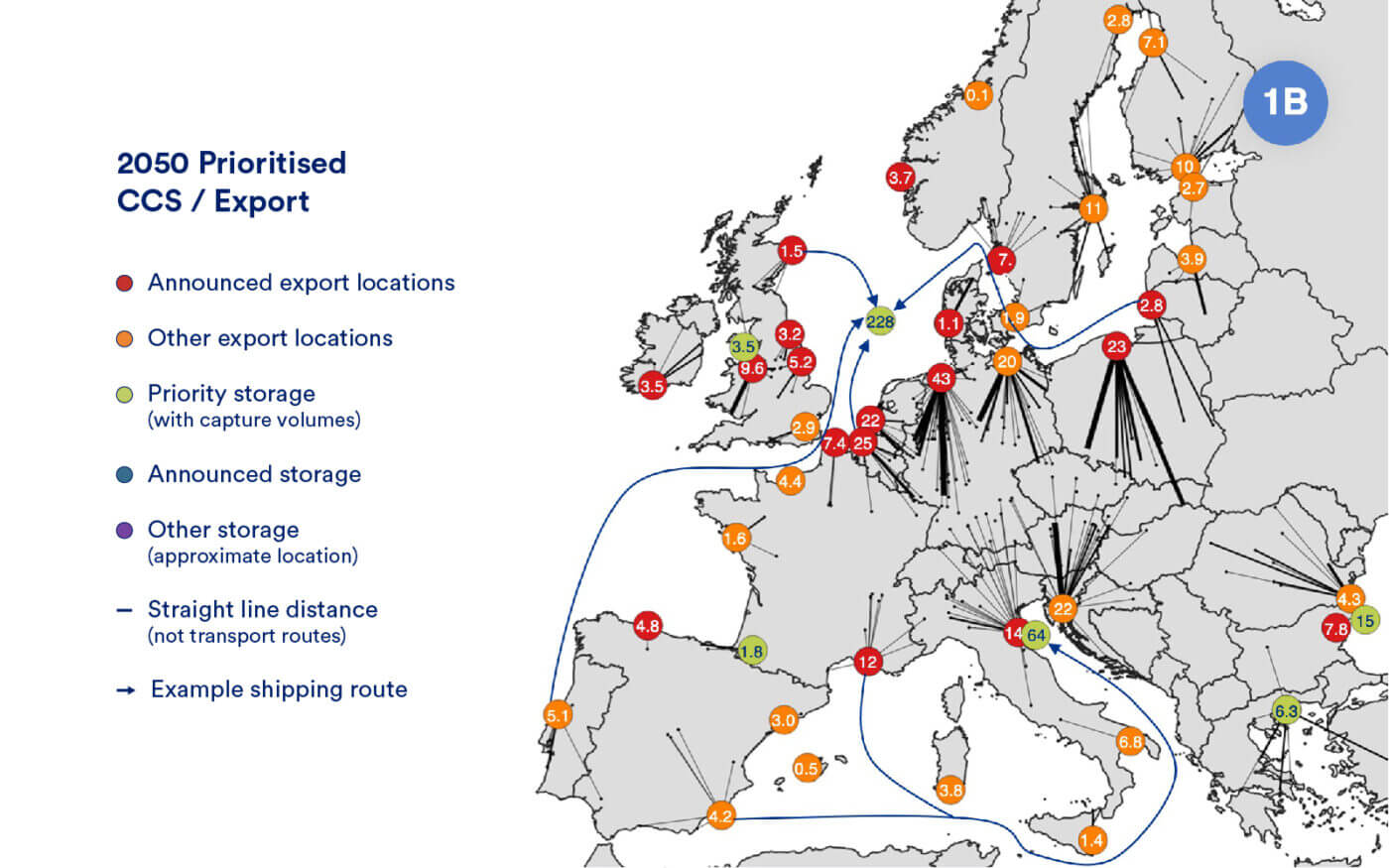
In the 2050 Prioritised CCS / Export scenario additional onshore transport networks need to be developed in Poland, Italy and Croatia by 2050
The development of more export locations does not significantly reduce transport distances.
- By 2050 onshore networks should be established to Rijeka (Croatia) and in eastern Germany to the Rostock hub to reduce the transport costs for individual emitters by exploiting economies of scale.
- Total onshore transport distance between emitters and export points for this scenario is 81,600 km, giving a total onshore CAPEX of €4.1-7.5 bn up to 2050 and an onshore OPEX for 2050 of €0.41-0.75 billion/year1 – assuming onshore transport is dominated by pipelines.
- An additional €6.1-22.8 billion is required in CAPEX for offshore transportation to key storage basins plus an OPEX of €2.04-4.81 billion/year in 20501 – assuming a mix of shipping and offshore pipelines is used.
Footnotes
- Element Energy – CO2 Shipping Model for BEIS for transport along 100 km long onshore pipelines at a rate of 0.5-2 MtCO2/year, 200 km offshore pipelines between 0.6-10 MtCO2/year and/or 500 km via ships at a rate of 0.5-9 MtCO2/year.
Credits
About the Authors
This report has been prepared by Element Energy, an ERM Group company.
Element Energy is a strategic energy consultancy, specialising in the intelligent analysis of low carbon energy. The team of over 100 specialists provides consultancy services across a wide range of sectors, including the built environment, carbon capture and storage, industrial decarbonisation, smart electricity and gas networks, energy storage, renewable energy systems and low carbon transport. Element Energy provides insights on both technical and strategic issues, believing that the technical and engineering understanding of the real world challenges support the strategic work. In June 2021, Element Energy joined the ERM Group, the largest independent sustainability consultancy, with a global footprint and over 7,000 employees worldwide.
This work was commissioned by Clean Air Task Force (CATF).
Clean Air Task Force (CATF) is a global nonprofit organization working to safeguard against the worst impacts of climate change by catalysing the rapid development and deployment of low-carbon energy and other climate-protecting technologies. With 25 years of internationally recognized expertise on climate policy and a fierce commitment to exploring all potential solutions, CATF is a pragmatic, non-ideological advocacy group with the bold ideas needed to address climate change. CATF has offices in Boston, Washington D.C., and Brussels, with staff working virtually around the world.
Authors
Silvian Baltac, Associate Partner
Elian Pusceddu, Principal Consultant
Conor O’Sullivan, Senior Consultant
Hannah Galbraith-Olive, Consultant
Cameron Henderson, Consultant
For comments or queries, please contact the authors at: [email protected]
Disclaimer
This study was commissioned by CATF. The conclusions and recommendations do not necessarily represent the view of CATF. Whilst every effort has been made to ensure the accuracy of this report, neither CATF nor Element Energy warrant its accuracy or will, regardless of its or their negligence, assume liability for any foreseeable or unforeseeable use made of this report which liability is hereby excluded.
In the 2035 Prioritised CCS / Domestic scenario, nearly half of emissions are still exported
The Domestic scenario includes more storage locations, but in 2035 these are limited to already announced / pilot projects.
- Announced export locations are included for those regions which have not yet developed local storage sites.
- Other export locations are also included based on demand from emitters.
Most emissions are stored in the North Sea, but there are potential capacity limitations in the Dutch sector.
- Total emissions captured in this scenario are 94 MtCO2/year, 66% of which (62 MtCO2/year) will ultimately be stored in the North Sea. This will allow 650-5800 years of theoretical storage in the North Sea if 2035 capture rates were maintained.
- However, if the emissions allocated to Dutch capture projects (9.3 MtCO2/year), were to only be stored in the Dutch storage sites, this would allow just 50-400 years of theoretical storage in the Dutch North Sea sector.
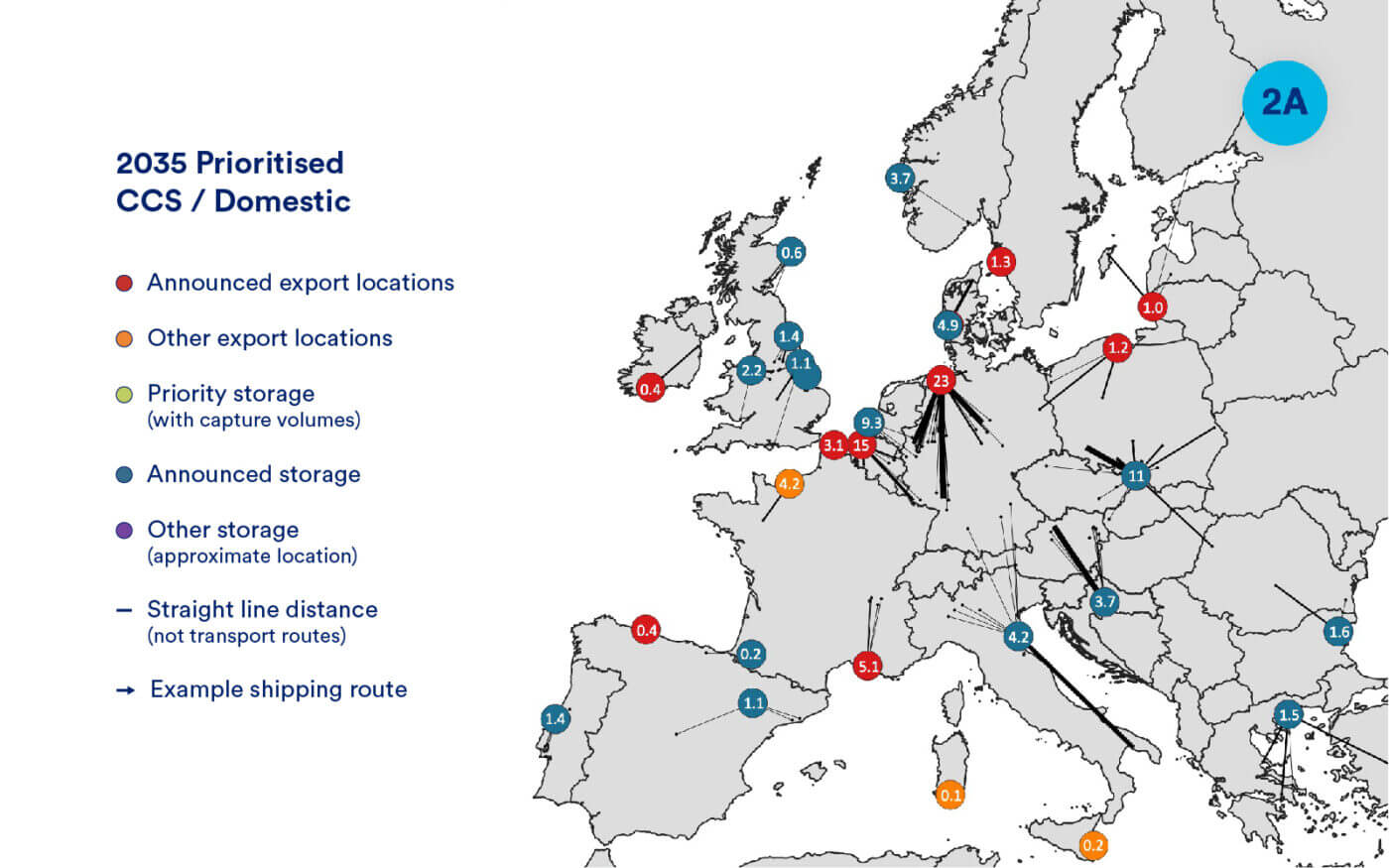
In the 2035 Prioritised CCS / Domestic scenario, nearly half of emissions are still exported
Transport distances are greatly reduced relative to the export scenario.
- Onshore transport required to transport emissions to domestic storage sites or export locations would cost €1.2-2.3 billion in CAPEX up to 2035 and €0.12-0.23 billion/year in OPEX for 20351.
- However, 47% of emissions are connected to an export point, rather than directly to a storage site because the region has not yet developed domestic storage, so further offshore transportation will be required for these emissions.
- This will cost €1.4-6.3 billion in CAPEX up to 2035 and €0.23-0.78 billion/year in OPEX for 20351.
- The total CAPEX for this scenario is €2.6-8.5 billion: €0.97-3.9 billion less than the 2035 Prioritised CCS/Export scenario since less additional offshore transport is required.
Footnotes
- Element Energy – CO2 Shipping Model for BEIS for transport along 100 km long onshore pipelines at a rate of 0.5-2 MtCO2/year, 200 km offshore pipelines between 0.6-10 MtCO2/year and/or 500 km via ships at a rate of 0.5-9 MtCO2/year.
Credits
About the Authors
This report has been prepared by Element Energy, an ERM Group company.
Element Energy is a strategic energy consultancy, specialising in the intelligent analysis of low carbon energy. The team of over 100 specialists provides consultancy services across a wide range of sectors, including the built environment, carbon capture and storage, industrial decarbonisation, smart electricity and gas networks, energy storage, renewable energy systems and low carbon transport. Element Energy provides insights on both technical and strategic issues, believing that the technical and engineering understanding of the real world challenges support the strategic work. In June 2021, Element Energy joined the ERM Group, the largest independent sustainability consultancy, with a global footprint and over 7,000 employees worldwide.
This work was commissioned by Clean Air Task Force (CATF).
Clean Air Task Force (CATF) is a global nonprofit organization working to safeguard against the worst impacts of climate change by catalysing the rapid development and deployment of low-carbon energy and other climate-protecting technologies. With 25 years of internationally recognized expertise on climate policy and a fierce commitment to exploring all potential solutions, CATF is a pragmatic, non-ideological advocacy group with the bold ideas needed to address climate change. CATF has offices in Boston, Washington D.C., and Brussels, with staff working virtually around the world.
Authors
Silvian Baltac, Associate Partner
Elian Pusceddu, Principal Consultant
Conor O’Sullivan, Senior Consultant
Hannah Galbraith-Olive, Consultant
Cameron Henderson, Consultant
For comments or queries, please contact the authors at: [email protected]
Disclaimer
This study was commissioned by CATF. The conclusions and recommendations do not necessarily represent the view of CATF. Whilst every effort has been made to ensure the accuracy of this report, neither CATF nor Element Energy warrant its accuracy or will, regardless of its or their negligence, assume liability for any foreseeable or unforeseeable use made of this report which liability is hereby excluded.
Widespread storage would more than halve transport CAPEX costs, but storage capacity may be limiting in the German North Sea and Switzerland in the 2050 Prioritised CCS / Domestic scenario
By 2050, more domestic storage has been developed, thus reducing the need for export locations.
- Some export locations remain, such as in Finland which lacks access to its own geological CO2 storage capacity.
- The development of onshore storage sites relies on a favourable political climate in each country.
Limited offshore storage capacity in the German North Sea means export to an alternative storage site may be more suitable.
- The storage site proposed in the German North Sea is allocated 31 MtCO2 /year in this scenario: this storage area will last for just 90-500 years based on theoretical storage capacities.
- A proposed storage site in Switzerland is also allocated 22 MtCO2 /year in this scenario and given the limited theoretical storage capacity in this region, this could last only 120 years.
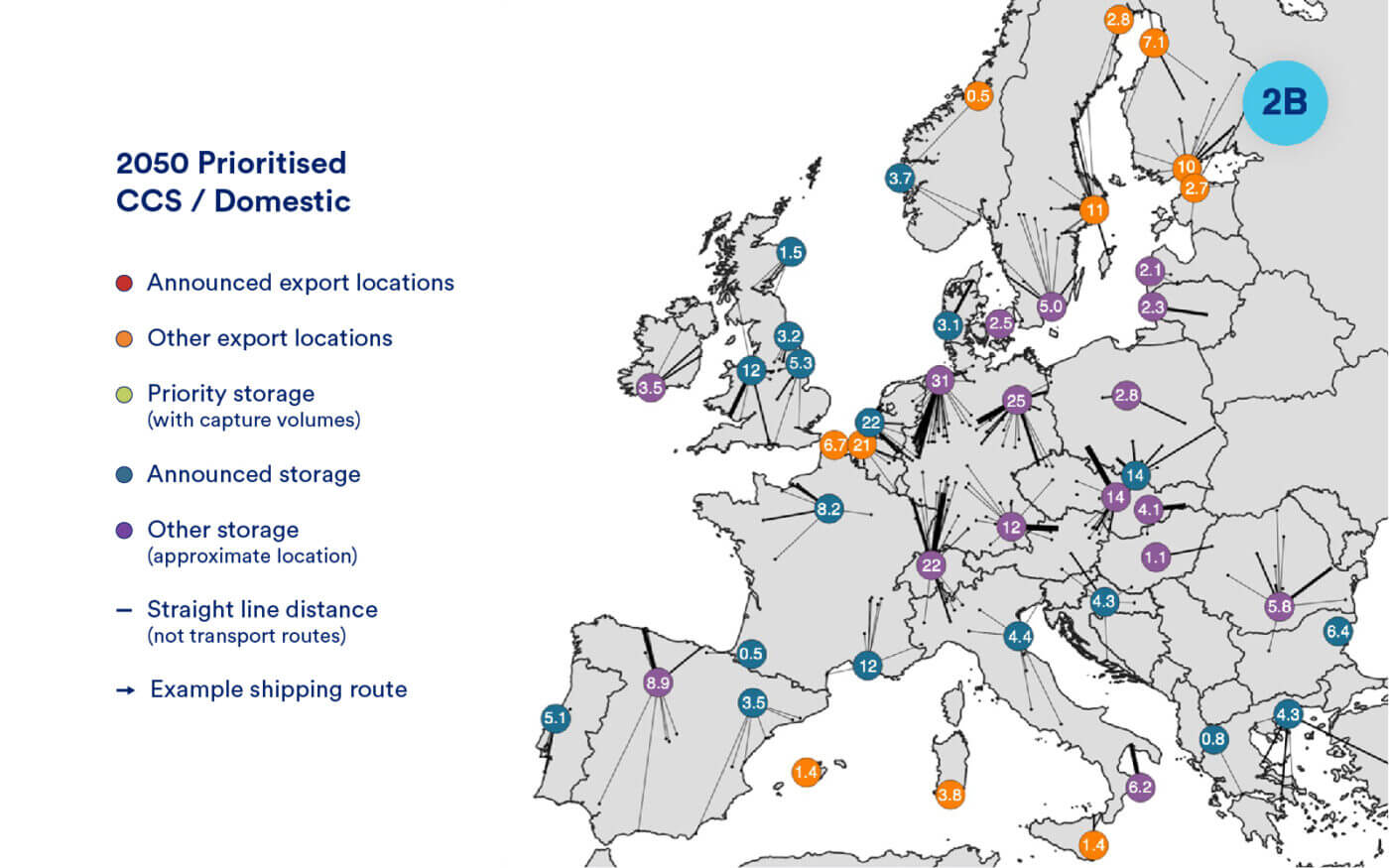
Widespread storage would more than halve transport CAPEX costs, but storage capacity may be limiting in the German North Sea and Switzerland in the 2050 Prioritised CCS / Domestic scenario
In the domestic scenario, savings can be made by cutting the requirement for onwards transport from an export point.
- The CAPEX of onshore transport is €4.1-7.5 billion in this scenario, with €0.48-0.75 billion for OPEX in the year 20501.
- However, 25% of emissions are transported via an export point in this scenario. This will add an additional €1.1-3.8 billion in CAPEX costs up to 2050 and €0.22-0.9 billion in OPEX for overseas transportation.
- Therefore, the total CAPEX of this scenario is €5.1-11.3 billion: between a half and a third of the total CAPEX of the 2050 Prioritised CCS / Export scenario.
Footnotes
- Element Energy – CO2 Shipping Model for BEIS for transport along 100 km long onshore pipelines at a rate of 0.5-2 MtCO2/year, 200 km offshore pipelines between 0.6-10 MtCO2/year and/or 500 km via ships at a rate of 0.5-9 MtCO2/year.
Credits
About the Authors
This report has been prepared by Element Energy, an ERM Group company.
Element Energy is a strategic energy consultancy, specialising in the intelligent analysis of low carbon energy. The team of over 100 specialists provides consultancy services across a wide range of sectors, including the built environment, carbon capture and storage, industrial decarbonisation, smart electricity and gas networks, energy storage, renewable energy systems and low carbon transport. Element Energy provides insights on both technical and strategic issues, believing that the technical and engineering understanding of the real world challenges support the strategic work. In June 2021, Element Energy joined the ERM Group, the largest independent sustainability consultancy, with a global footprint and over 7,000 employees worldwide.
This work was commissioned by Clean Air Task Force (CATF).
Clean Air Task Force (CATF) is a global nonprofit organization working to safeguard against the worst impacts of climate change by catalysing the rapid development and deployment of low-carbon energy and other climate-protecting technologies. With 25 years of internationally recognized expertise on climate policy and a fierce commitment to exploring all potential solutions, CATF is a pragmatic, non-ideological advocacy group with the bold ideas needed to address climate change. CATF has offices in Boston, Washington D.C., and Brussels, with staff working virtually around the world.
Authors
Silvian Baltac, Associate Partner
Elian Pusceddu, Principal Consultant
Conor O’Sullivan, Senior Consultant
Hannah Galbraith-Olive, Consultant
Cameron Henderson, Consultant
For comments or queries, please contact the authors at: [email protected]
Disclaimer
This study was commissioned by CATF. The conclusions and recommendations do not necessarily represent the view of CATF. Whilst every effort has been made to ensure the accuracy of this report, neither CATF nor Element Energy warrant its accuracy or will, regardless of its or their negligence, assume liability for any foreseeable or unforeseeable use made of this report which liability is hereby excluded.
In the 2035 Technical Potential / Export scenario, if storage is limited to a few key basins, storage in Northern Italy is at risk of reaching capacity
The Technical Potential scenario represents the upper limit of CCS deployment in Europe.
- This carbon capture scenario represents the 2035 timestep between today and the technical limit of CCS being achieved in 2050.
- Storage is limited to a few key basins, including the North Sea.
Most emissions are stored in the North Sea, but storage sites in Northern Italy are at risk of reaching a capacity limit in this scenario.
- 74% of emissions captured (123 MtCO2/year) in this scenario are stored in the North Sea after being aggregated at announced and other priority export locations. This gives 330-3000 years of theoretical storage capacity.
- 32 MtCO2/year would be stored in Northern Italy, which would have a minimum lifetime of just 46 years if the low theoretical storage capacity estimate is considered for Northern Italy.*
- It would be advisable for some Mediterranean emissions to be shipped further to the Aegean/Black Seas or emitters around the Alps could be transported northwards instead of connecting to an export point in Northern Italy.
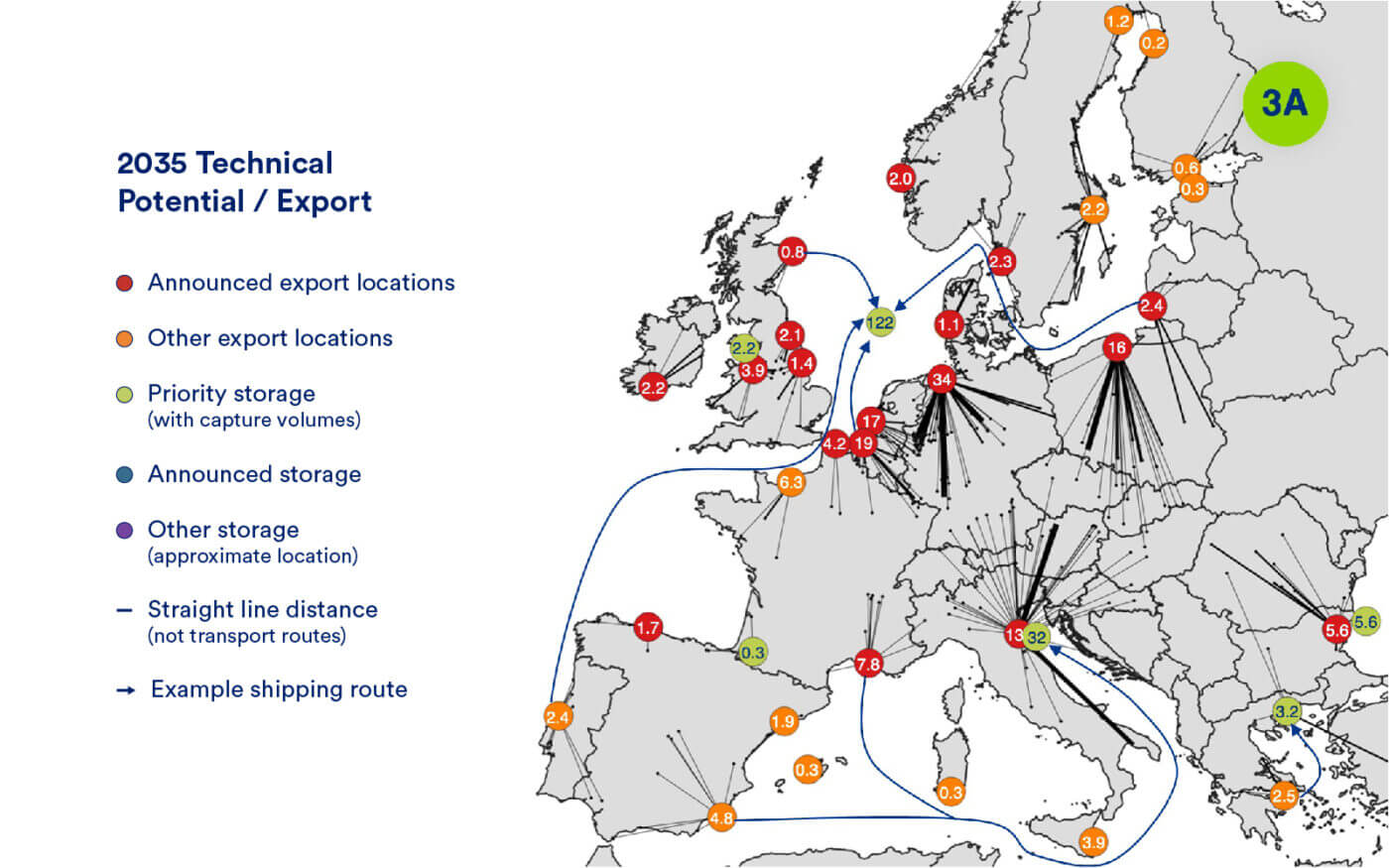
In the 2035 Technical Potential / Export scenario, if storage is limited to a few key basins, storage in Northern Italy is at risk of reaching capacity
Transport distances are much further in the export scenario compared to domestic storage development.
- Overland transport is required to transport emissions to export locations, covering a total distance of 93,100 km.
- Assuming pipeline transport, the total overland CAPEX would be €2.2-4.0 billion up to 2035 and OPEX is €0.2-0.4 billion/year in 20351.
- Further transport will be required in this scenario to access storage capacity. This could add between €1.9-15.8 billion in CAPEX and €0.95-2.5 billion/year in OPEX depending on flow rate1.
- However, some of these export points (e.g., UK clusters, Ravenna) will use offshore pipelines whereas some (e.g., from Germany / Belgium / Netherlands) may use a combination of shipping and offshore pipelines and some may be totally reliant on shipping (e.g., Northern Sweden/Finland) to store CO2 in the North Sea.
Footnotes
- Element Energy – CO2 Shipping Model for BEIS for transport along 100 km long onshore pipelines at a rate of 0.5-2 MtCO2/year, 200 km offshore pipelines between 0.6-10 MtCO2/year and/or 500 km via ships at a rate of 0.5-9 MtCO2/year.
Credits
About the Authors
This report has been prepared by Element Energy, an ERM Group company.
Element Energy is a strategic energy consultancy, specialising in the intelligent analysis of low carbon energy. The team of over 100 specialists provides consultancy services across a wide range of sectors, including the built environment, carbon capture and storage, industrial decarbonisation, smart electricity and gas networks, energy storage, renewable energy systems and low carbon transport. Element Energy provides insights on both technical and strategic issues, believing that the technical and engineering understanding of the real world challenges support the strategic work. In June 2021, Element Energy joined the ERM Group, the largest independent sustainability consultancy, with a global footprint and over 7,000 employees worldwide.
This work was commissioned by Clean Air Task Force (CATF).
Clean Air Task Force (CATF) is a global nonprofit organization working to safeguard against the worst impacts of climate change by catalysing the rapid development and deployment of low-carbon energy and other climate-protecting technologies. With 25 years of internationally recognized expertise on climate policy and a fierce commitment to exploring all potential solutions, CATF is a pragmatic, non-ideological advocacy group with the bold ideas needed to address climate change. CATF has offices in Boston, Washington D.C., and Brussels, with staff working virtually around the world.
Authors
Silvian Baltac, Associate Partner
Elian Pusceddu, Principal Consultant
Conor O’Sullivan, Senior Consultant
Hannah Galbraith-Olive, Consultant
Cameron Henderson, Consultant
For comments or queries, please contact the authors at: [email protected]
Disclaimer
This study was commissioned by CATF. The conclusions and recommendations do not necessarily represent the view of CATF. Whilst every effort has been made to ensure the accuracy of this report, neither CATF nor Element Energy warrant its accuracy or will, regardless of its or their negligence, assume liability for any foreseeable or unforeseeable use made of this report which liability is hereby excluded.
Even when >2/3rds of CO2 emissions in the 2050 Technical Potential / Export scenario are
stored in the North Sea, there is still >120 years worth of theoretical storage capacity
By 2050, many more export locations have been developed to serve coastline emitters wanting to use CCS to decarbonise.
- These include locations around the Baltic Sea such as Rostock in Germany and in the Mediterranean in southern Italy.
- Although more export points have been developed, inland emitters will still need to transport their emissions significant distances overland before they have to be further transported to their final storage site.
Storage in the Mediterranean needs to be developed beyond Northern Italy to ensure capacity is not reached.
- More emissions are captured and stored in the North Sea (346 MtCO2/year), but it accounts for a lower proportion (67%) of total emissions in this scenario. This gives 120-1000 years theoretical storage capacity.
- 128 MtCO2/year would be captured in the Northern Italy region. This region could have a minimum lifetime of just 11 years if low theoretical storage capacity estimates are considered*.
- In 2050, it would still be advisable for some Mediterranean emissions to be shipped further to the Aegean/Black Seas or emitters around the Alps could be transported northwards instead of connecting to an export point in Northern Italy.
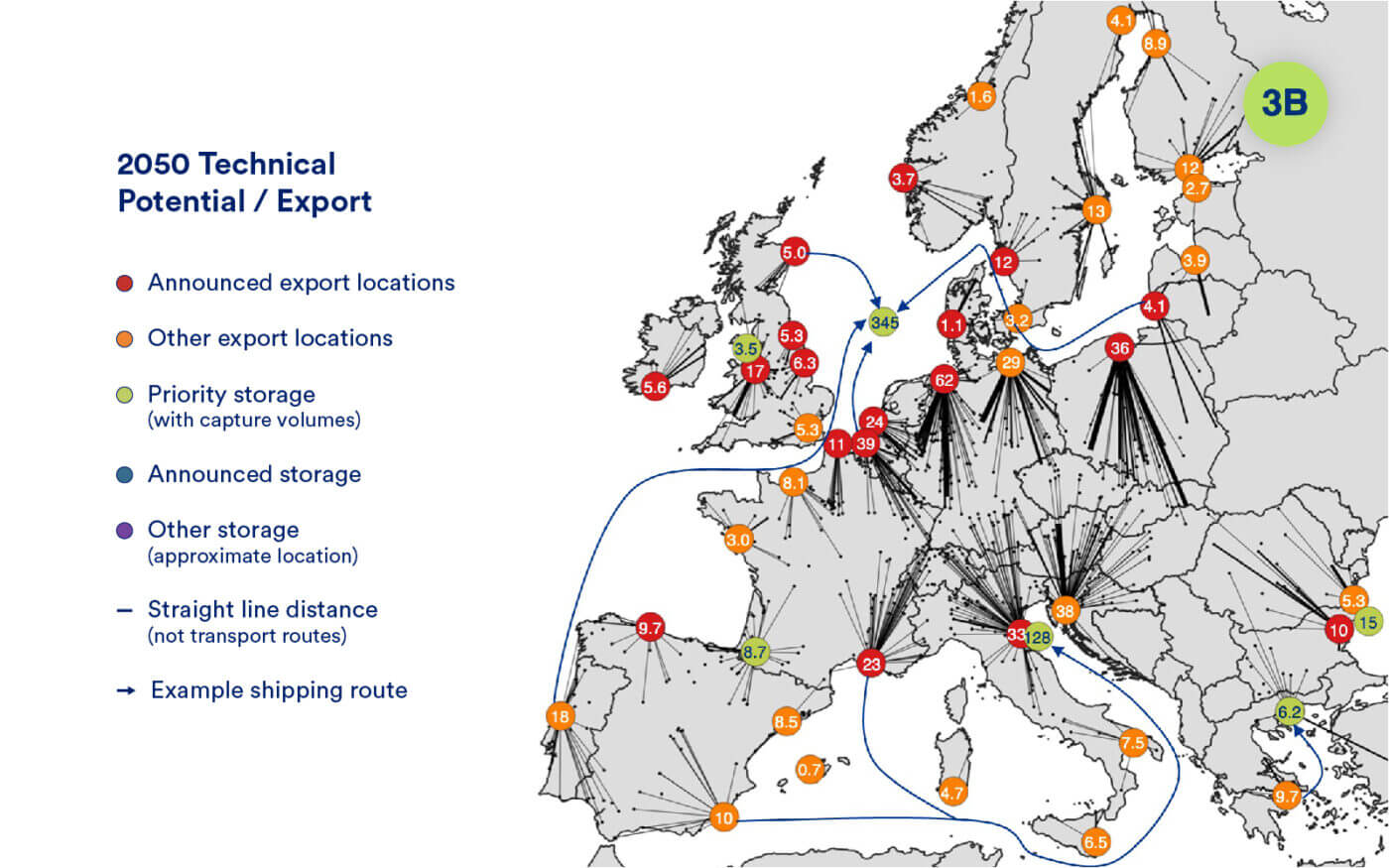
Even when >2/3rds of CO2 emissions in the 2050 Technical Potential / Export scenario are stored in the North Sea, there is still >120 years worth of theoretical storage capacity
Average transport distances increase as dispersed emitters seek to use CCS to decarbonise.
- The total distance between emitters and export points increases to 99,300 km in this scenario, increasing onshore CAPEX transport costs to €7.0-13.0 billion – an increase of €3.0-5.5 billion compared to the 2050 Prioritised CCS Potential/Export scenario1.
- Additional costs will be incurred due to transport of CO2 from an export point to its final storage location, which will be between €10.3-37.9 billion in CAPEX and €3.6-8.3 billion/year in OPEX for this scenario.
Footnotes
- Element Energy – CO2 Shipping Model for BEIS for transport along 100 km long onshore pipelines at a rate of 0.5-2 MtCO2/year, 200 km offshore pipelines between 0.6-10 MtCO2/year and/or 500 km via ships at a rate of 0.5-9 MtCO2/year.
Credits
About the Authors
This report has been prepared by Element Energy, an ERM Group company.
Element Energy is a strategic energy consultancy, specialising in the intelligent analysis of low carbon energy. The team of over 100 specialists provides consultancy services across a wide range of sectors, including the built environment, carbon capture and storage, industrial decarbonisation, smart electricity and gas networks, energy storage, renewable energy systems and low carbon transport. Element Energy provides insights on both technical and strategic issues, believing that the technical and engineering understanding of the real world challenges support the strategic work. In June 2021, Element Energy joined the ERM Group, the largest independent sustainability consultancy, with a global footprint and over 7,000 employees worldwide.
This work was commissioned by Clean Air Task Force (CATF).
Clean Air Task Force (CATF) is a global nonprofit organization working to safeguard against the worst impacts of climate change by catalysing the rapid development and deployment of low-carbon energy and other climate-protecting technologies. With 25 years of internationally recognized expertise on climate policy and a fierce commitment to exploring all potential solutions, CATF is a pragmatic, non-ideological advocacy group with the bold ideas needed to address climate change. CATF has offices in Boston, Washington D.C., and Brussels, with staff working virtually around the world.
Authors
Silvian Baltac, Associate Partner
Elian Pusceddu, Principal Consultant
Conor O’Sullivan, Senior Consultant
Hannah Galbraith-Olive, Consultant
Cameron Henderson, Consultant
For comments or queries, please contact the authors at: [email protected]
Disclaimer
This study was commissioned by CATF. The conclusions and recommendations do not necessarily represent the view of CATF. Whilst every effort has been made to ensure the accuracy of this report, neither CATF nor Element Energy warrant its accuracy or will, regardless of its or their negligence, assume liability for any foreseeable or unforeseeable use made of this report which liability is hereby excluded.
Even by 2035 in the Technical Potential / Domestic scenario, the development of strategic
domestic storage sites significantly reduces the total CAPEX costs of CO2 transport
In 2035, only announced export and storage locations have been developed, along with a few key export locations in the Baltic region.
- Announced export locations are included for those regions which have not yet developed local storage sites.
Key development opportunities include an onshore pipeline network in Germany and onshore storage in southern Poland.
- Development of an onshore pipeline network in Germany connected to the hub in Wilhelmshaven would be beneficial, as 34 MtCO2/year is transported through this export location to be stored in the North Sea.
- Onshore storage in the Upper Silesia region of Poland is allocated 15 MtCO2/year of emissions. Assuming all of this is injected at a constant rate, given storage capacity in southern Poland, the storage would last for a minimum of 147 years in the low storage capacity scenario.
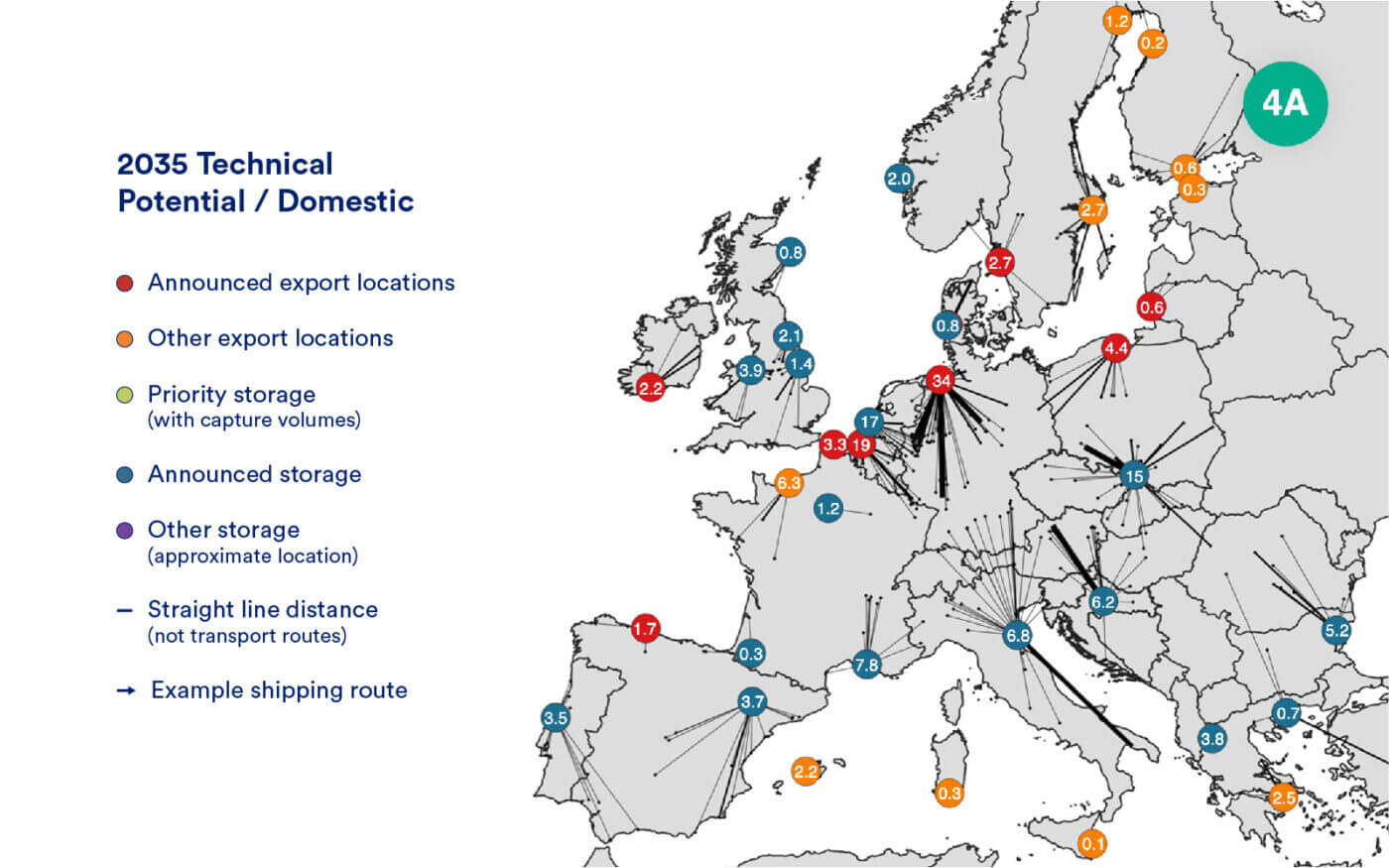
Even by 2035 in the Technical Potential / Domestic scenario, the development of strategic
domestic storage sites significantly reduces the total CAPEX costs of CO2 transport
Reliance on export locations in 2035 adds to the transport component of the CCS value chain.
- The total transport distance required to move emissions directly to storage locations is 38,800 km in this scenario.
- If pipeline transport was used as the onshore transport medium, this scenario would cost €2.2-4 billion in CAPEX and €0.22-4.0 billion/year in OPEX1.
- However, 53% of emissions are connected to an export point, rather than directly to a storage site because the region has not yet developed domestic storage.
- Further transport will be needed to access storage capacity; this would cost €2.2-4.5 billion in CAPEX and €0.45-1.3 billion/year in OPEX1.
- The total CAPEX cost for this scenario is €4.3-13.0 billion: €1.7-6.8 billion less than for the 2035 Technical Potential / Export scenario.
Footnotes
- Element Energy – CO2 Shipping Model for BEIS for transport along 100 km long onshore pipelines at a rate of 0.5-2 MtCO2/year, 200 km offshore pipelines between 0.6-10 MtCO2/year and/or 500 km via ships at a rate of 0.5-9 MtCO2/year.
Credits
About the Authors
This report has been prepared by Element Energy, an ERM Group company.
Element Energy is a strategic energy consultancy, specialising in the intelligent analysis of low carbon energy. The team of over 100 specialists provides consultancy services across a wide range of sectors, including the built environment, carbon capture and storage, industrial decarbonisation, smart electricity and gas networks, energy storage, renewable energy systems and low carbon transport. Element Energy provides insights on both technical and strategic issues, believing that the technical and engineering understanding of the real world challenges support the strategic work. In June 2021, Element Energy joined the ERM Group, the largest independent sustainability consultancy, with a global footprint and over 7,000 employees worldwide.
This work was commissioned by Clean Air Task Force (CATF).
Clean Air Task Force (CATF) is a global nonprofit organization working to safeguard against the worst impacts of climate change by catalysing the rapid development and deployment of low-carbon energy and other climate-protecting technologies. With 25 years of internationally recognized expertise on climate policy and a fierce commitment to exploring all potential solutions, CATF is a pragmatic, non-ideological advocacy group with the bold ideas needed to address climate change. CATF has offices in Boston, Washington D.C., and Brussels, with staff working virtually around the world.
Authors
Silvian Baltac, Associate Partner
Elian Pusceddu, Principal Consultant
Conor O’Sullivan, Senior Consultant
Hannah Galbraith-Olive, Consultant
Cameron Henderson, Consultant
For comments or queries, please contact the authors at: [email protected]
Disclaimer
This study was commissioned by CATF. The conclusions and recommendations do not necessarily represent the view of CATF. Whilst every effort has been made to ensure the accuracy of this report, neither CATF nor Element Energy warrant its accuracy or will, regardless of its or their negligence, assume liability for any foreseeable or unforeseeable use made of this report which liability is hereby excluded.
Widespread development of storage sites will reduce CO2 transport costs as average transport distance is reduced in the 2050 Technical Potential / Domestic scenario
By 2050, every country able to has developed a storage location, although countries such as Finland must still rely on exports.
- Some countries (e.g., UK, France, Germany) have developed multiple storage locations in different regions.
- It is assumed no political barriers exist to the location of onshore storage and its development.
Limitations in storage capacity occur offshore Germany, in Switzerland and in the Netherlands.
- 49 MtCO2/year is allocated to the German North Sea. In this scenario, there will only be capacity to store captured emissions for a minimum of 60 years in the low storage scenario.
- A similar situation occurs in Switzerland, which receives 38 MtCO2/year and has a minimum of 70 years storage capacity.
- Both these areas should consider building a transport network so captured emissions can be stored elsewhere if required.
- The Dutch North Sea storage is allocated 24 MtCO2/year in this scenario, but at a high storage capacity estimate of 3600 Mt, this could last for a maximum of 150 years. However, these emissions could be relatively easily exported to an alternative storage location.
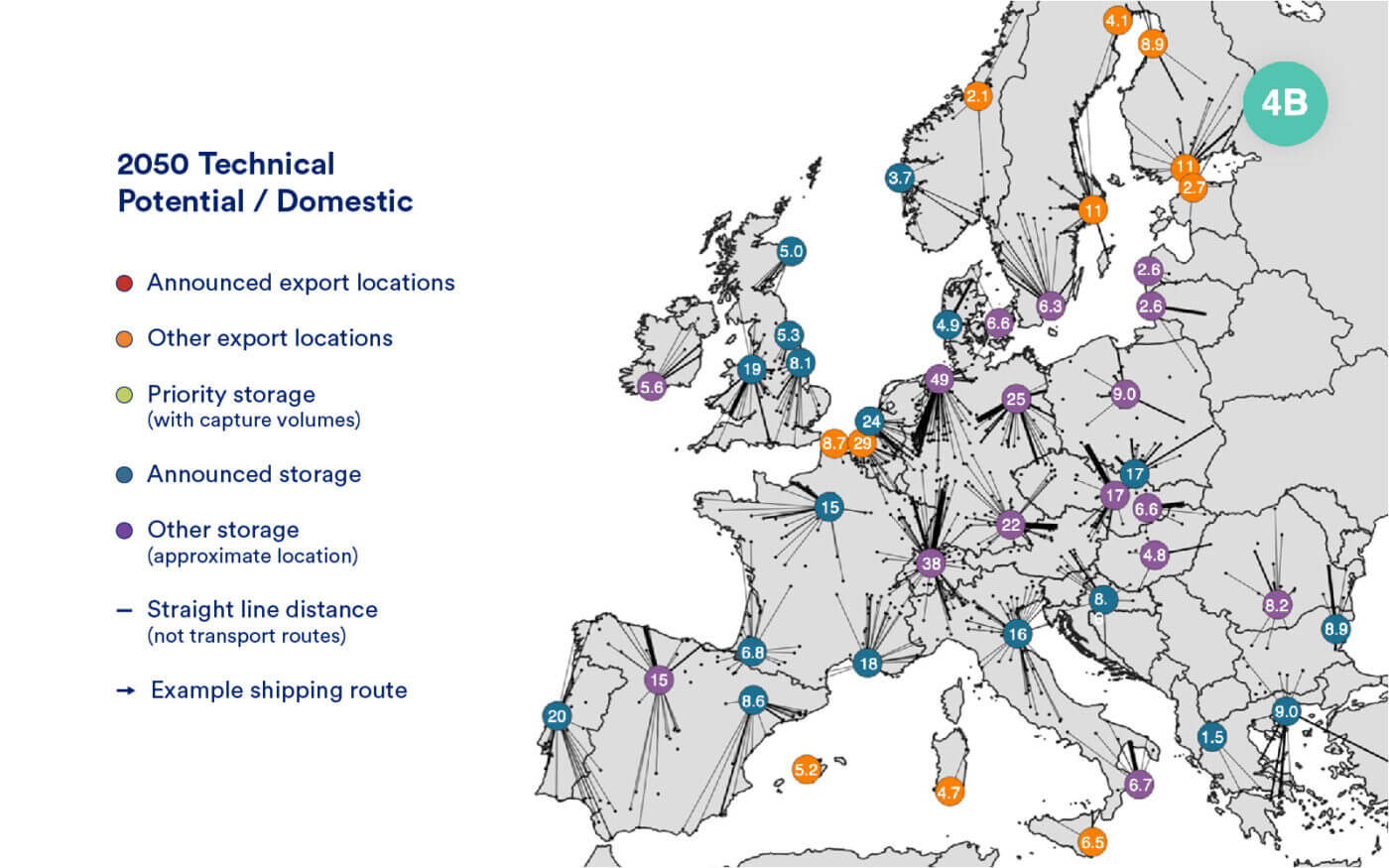
Widespread development of storage sites will reduce CO2 transport costs as average transport distance is reduced in the 2050 Technical Potential / Domestic scenario
Given the expansion in onshore storage locations, transport costs are lower than in an export dominant.
- 81% of emissions are connected to a storage site by 2050 in this scenario – a 28% increase from 2035 as more storage sites are developed.
- Compared to the 2050 Prioritised CCS / Domestic scenario, overall CAPEX costs are increased by €3.7-8.1 billion – reaching a total of €8.9-19.4 billion1.
Footnotes
- Element Energy – CO2 Shipping Model for BEIS for transport along 100 km long onshore pipelines at a rate of 0.5-2 MtCO2/year, 200 km offshore pipelines between 0.6-10 MtCO2/year and/or 500 km via ships at a rate of 0.5-9 MtCO2/year.
Credits
About the Authors
This report has been prepared by Element Energy, an ERM Group company.
Element Energy is a strategic energy consultancy, specialising in the intelligent analysis of low carbon energy. The team of over 100 specialists provides consultancy services across a wide range of sectors, including the built environment, carbon capture and storage, industrial decarbonisation, smart electricity and gas networks, energy storage, renewable energy systems and low carbon transport. Element Energy provides insights on both technical and strategic issues, believing that the technical and engineering understanding of the real world challenges support the strategic work. In June 2021, Element Energy joined the ERM Group, the largest independent sustainability consultancy, with a global footprint and over 7,000 employees worldwide.
This work was commissioned by Clean Air Task Force (CATF).
Clean Air Task Force (CATF) is a global nonprofit organization working to safeguard against the worst impacts of climate change by catalysing the rapid development and deployment of low-carbon energy and other climate-protecting technologies. With 25 years of internationally recognized expertise on climate policy and a fierce commitment to exploring all potential solutions, CATF is a pragmatic, non-ideological advocacy group with the bold ideas needed to address climate change. CATF has offices in Boston, Washington D.C., and Brussels, with staff working virtually around the world.
Authors
Silvian Baltac, Associate Partner
Elian Pusceddu, Principal Consultant
Conor O’Sullivan, Senior Consultant
Hannah Galbraith-Olive, Consultant
Cameron Henderson, Consultant
For comments or queries, please contact the authors at: [email protected]
Disclaimer
This study was commissioned by CATF. The conclusions and recommendations do not necessarily represent the view of CATF. Whilst every effort has been made to ensure the accuracy of this report, neither CATF nor Element Energy warrant its accuracy or will, regardless of its or their negligence, assume liability for any foreseeable or unforeseeable use made of this report which liability is hereby excluded.
The development of CO2 transport networks across Europe will require significant investment up to €2.6-50.9 billion in CAPEX
Each scenario (1a-4b) represents a range of transport options for different industrial demand and storage capacity scenarios.
- The Prioritised CCS scenarios represents the upper end of literature estimates on CCS uptake for each industrial sector whereas the Technical Potential scenarios aims to test the limits of geological storage capacity.
- In the Export scenario only a few key storage basins are developed, whereas in the Domestic scenario, widespread storage is modelled.
- By combining these scenarios, a range of transport options for CO2 across Europe have been developed.
Domestic scenarios are cheaper than Export scenarios in terms of both CAPEX and OPEX.
- An export-heavy solution to CO2 transport networks gives more flexibility to emitters as to where their emissions can be stored.
These CAPEX and OPEX costs represent a high estimate for CCS transport costs across Europe.
- CCS is likely to be a high-cost decarbonisation option only used in hard-to-abate industries, although economies of scale – particularly collaborating with nearby emitters – will increase the attractiveness of CCS as a decarbonisation option for certain emitters.
- Notwithstanding, significant investments will need to be made in CCS transport networks to incentivise decarbonisation of these hard-to-abate industries.
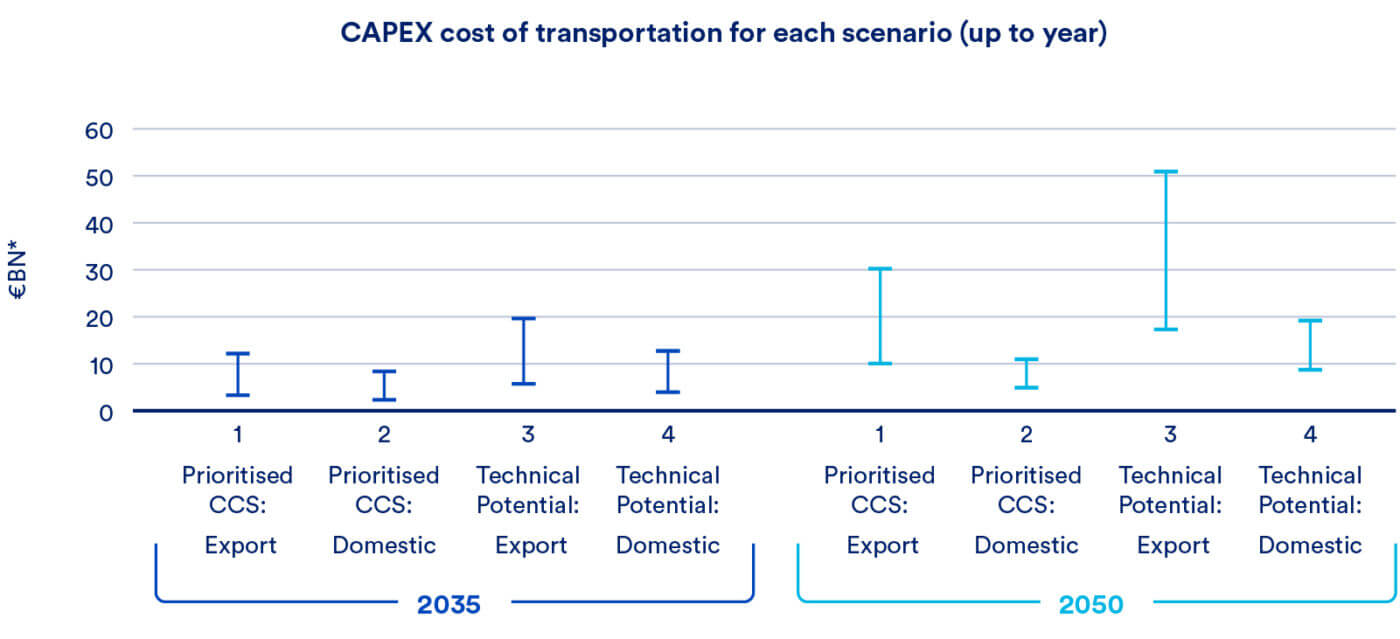
Conclusions and Recommendations
- Europe as a whole has plenty of theoretical storage capacity for long-term CO2 storage to last at least 130 years even if the technical limit of CO2 emissions from both industrial and non-industrial sources is considered.
- Most countries have sufficient storage capacities to at least store their own emissions but countries which may not have enough storage capacity include (but are not necessarily limited to), the Netherlands, Austria, Belgium, Estonia, Finland and Luxembourg.
- Current identified storage capacity in announced projects is not sufficient to store potential CO2 demand in 2035. Countries should seek to identify multiple storage locations to ensure the long-term viability of not only their announced storage projects, but also CCS across Europe. More storage basins should be developed across Europe in order to match potential demand.
- Regions of high emissions such as North-West Germany and Poland would particularly benefit from either onshore storage development or establishing an onshore pipeline network, or an alternatives such as barge shipping or trains, to certain export points in the near future (i.e., by 2035).
- By 2035, export points need to be developed in regions far from storage sites (e.g., Finland, Western Mediterranean) if CCS is to be a viable decarbonisation option in these regions. Efforts should also be made to build a flexible, cross-border storage system in the North Sea, not only to improve resilience, but also to ensure all CO2 is stored safely and is not constrained by political boundaries.
- In all scenarios, CCS demand is concentrated in clusters where emissions density is high, with uptake at dispersed sites prioritised in the cement & lime and waste management sectors due to their reliance on CCS pathways to decarbonise.
- Non-industrial CCS demand could grow rapidly across Europe, accounting for an additional 700+ MtCO2/year by 2050. Negative emissions technologies (BECCS and DAC) could account for the greatest additional demand, however, high levels of uncertainty are associated with these forecasts.