Analysis of Lifecycle Greenhouse Gas Emissions of Natural Gas and Coal Powered Electricity
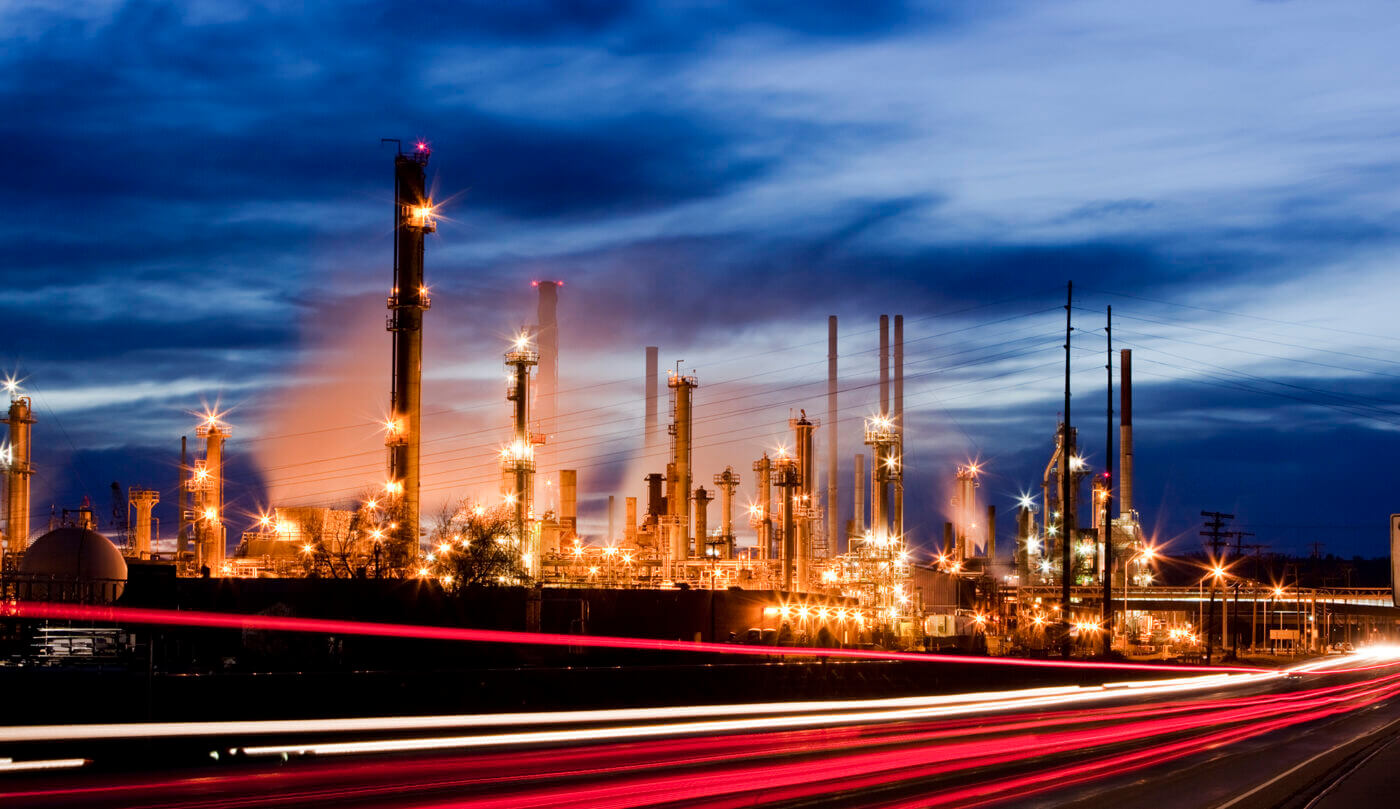
Zusammenfassung
- Although less than half of world gas consumption goes to electric power, most analyses of gas lifecycle GHG emissions, and specifically impacts of U.S. LNG exports, focus on the power sector. But determining the net climate impact of additional U.S. gas exports for electric power generation is complicated by a number of key variables, including the amount of methane and CO2 emissions from specific supply chains, whether the additional gas replaces coal or another energy source or is additive to existing energy sources, the source of potential alternative non-U.S. gas suppliers and their GHG footprints, downstream combustion efficiencies, and second order price and demand effects of additional U.S. supply. The multiplicity of factors makes definitive statements on real world GHG impacts highly problematic.
- Even in a simplified model, assuming one-to-one displacement of coal with gas power generation in developing countries, results can vary substantially due to the variation in the measured methane (CH4) emissions from some natural gas supply chains. In CATF’s LCA analysis of U.S. LNG shipments to Vietnam, for example, depending on upstream methane leakage rates, imported gas that facilitates coal-to-gas fuel switching could range from reducing power greenhouse gas impacts by as much as 40% to worsening them by 20%. Evidence does not show that U.S. gas is cleaner on average than average global gas, so it cannot be concluded as a general matter that exported U.S. LNG would either increase or decrease global GHG emissions; an analysis of specific U.S. sources and supply chains as well as the efficiency of downstream combustion would be required.
- CATF’s analysis further underscores the that, over the long run, to be compatible with stringent climate goals, CO2 and CH4 emissions from natural gas, whether locally produced or imported, must be maximally mitigated, including methane abatement for gas production and utilization of carbon capture and storage (CCS) for power plant CO2. Fortunately, we have low-cost options today to minimize the upstream climate impact of whatever gas is used, and there is no excuse for delaying deployment of these options.
- Introduction
- How do lifecycle emissions of U.S.-exported gas and exported coal used for power generation compare?
- Variation in upstream emissions of natural gas and coal
- Mitigation is critical in the short and long term, regardless of LCA analysis
- Schlussfolgerung
- Appendix A: Parameters used in lifecycle assessment analysis
- Appendix B: Results for cases presented in figures
Introduction
Increasing the export of liquified natural gas (LNG) from the United States has been hypothesized to have climate benefits based on the assumption that the LNG will displace coal for electricity generation.1 To investigate this claim, it is critical to first determine whether natural gas is indeed replacing coal, and then, assuming gas does replace coal, to compare the full lifecycle emissions of both coal and natural gas, including both upstream emissions (methane and carbon dioxide) and stack emissions for each fuel. The current analysis presents a simplified one-for-one electric power gas/coal displacement scenario and suggests how the answers vary widely depending on a variety of assumptions, and the assumed level of mitigation, upstream and downstream, is a significant part of the analysis.
Not surprisingly, this issue is highly politicized, and various models and studies come to very different conclusions depending on varied assumptions.
First, it is critical to understand what is the “but for” case if the U.S. gas is not exported. What would be the alternative energy source that would be realistically utilized at the end use, if any? And would other imported gas fill any gaps not provided by the exporter in question? Reporting on counterparty contracts with U.S. LNG companies suggests that recent export demand is driven by Europe and Asia, each location representing a quarter of demand, and the remaining half driven by companies who have a diverse portfolio of customers in different regions.2 Some analysts have suggested that a significant portion of the recent LNG expansion was driven by demand growth in developing nations, not by fuel switching, and that there exists potential for overcapacity in the future.3 Even for the known locations, the use of LNG exported gas and its impacts on local energy supply remain uncertain. Direct coal to gas switching may also be impeded because many places lack natural gas pipelines to bring LNG from the import terminal to where it is needed.4
Second, what are the second order effects of gas exports on U.S. gas consumption – e.g., would gas exports lead to higher U.S. prices, and potentially resulting lower demand, and how would this be offset by marginally lower global equilibrium prices? How will exports affect U.S. gas and oil production levels? U.S. LNG exports will likely produce knock-on effects that go beyond any one contract. Internationally, increased supply of lower cost gas could drive fuel switching from coal but also lower energy prices leading to increased energy consumption overall.5 Long-term market-impact studies suggest expanded U.S. LNG exports could produce a range of climate effects from limited benefits to adverse emission increases.6,7 A recent study estimated that market effects of reduced global gas prices may lower the benefits of coal to gas switching by 80%, with the 90% probability range of the impact spanning negative and positive emission impacts.8 In addition, reduced energy prices may lower the business case for zero-carbon electricity.9
Third, what are the long-term effects of gas imports, either in “locking in” unabated gas consumption for the future, or, alternatively, facilitating economic growth which enables gas-receiving countries to progress over time to higher levels of climate mitigation and transition to lower carbon energy sources?
Most models greatly simplify this complex set of issues by focusing on assumed direct displacement of one fuel source with another without considering multiple “but for” scenarios, second order, and long-term effects. Most focus on a single use case, i.e. displacement of coal-fired power generation with natural gas. This focus on the electric power sector is notable and highly incomplete since the vast majority of gas globally is used in industry (for combustion and feedstocks) and building usage, not power generation. Nevertheless, even assuming one-to-one displacement of coal with gas generation in developing countries, we find that exported gas may or may not be better for the climate than coal for electricity – given the magnitude of measured emissions from the natural gas supply chain and the variation in upstream emissions for natural gas from differing origins. The remainder of the analysis in this white paper investigates the comparison of coal and natural gas for several cases using a model developed by Clean Air Task Force.
How do lifecycle emissions of U.S.-exported gas and exported coal used for power generation compare?
Whether natural gas exported as LNG has lower emissions than coal when used as a fuel for power generation, when upstream emissions are taken into account, depends on many factors. The climate impact of burning a fuel for power generation includes the emissions associated with producing and transporting the fuel to the power plant, in addition to the “stack” emissions from the power station. For both coal- and natural gas-fired generation, total emissions are dominated by three categories of emissions: stack carbon dioxide from the power plant; “upstream” methane from production, processing, and transport of the gas or coal;10 and upstream CO2 from production, processing, and transport of the fuel. (For each case we discuss below, Appendix B lists emissions by segment.)
Methane, the main component of natural gas, warms the climate far more on a ton-for-ton basis than CO2, especially in the first decades after release. The twenty-year global warming potential methane (GWP) is 82.5, while the hundred-year GWP is about 30.11 This means that relatively small leaks or releases of methane from a fuel upstream of power plants will have a large impact on total lifecycle emissions from producing and using the fuel.
These factors have long been recognized and many lifecycle analyses have been published comparing the climate impacts of producing and using coal and gas to generate electricity. Many of these utilize EPA’s estimates of methane emissions from natural gas systems and coal production (directly or indirectly). However, it has long been recognized that EPA’s inventories substantially underestimate emissions of methane from natural gas systems.12 EPA’s inventories are based on emissions factors, typically representing emissions from equipment operating normally, and it is well documented that unintended emissions resulting from abnormal operations or malfunctions substantially increase real emissions from these systems. Because these unintended emissions are very difficult to predict or measure “at the source,” the most accurate assessment of emissions from oil and gas operations is done with “top-down” measurements that use measurements of methane in ambient air downwind of oil and gas sites. These measurements capture all emissions and can readily be performed at random times, allowing random sampling among sites, which is essential for capturing emissions from abnormal conditions and malfunctions.
The most comprehensive estimate of emissions from U.S. oil and gas systems, based on top-down measurements from thousands of sites across the oil and natural gas system, reported that methane emissions from these systems in 2015 were equivalent to 2.3% of the methane produced by the system.13 In this analysis, we adjust the 2.3% figure to account for the fact that some of these methane emissions should be attributed to oil rather than natural gas, and thus we use a leak rate of 1.8% for the U.S. national average.
We use this result as the basis of our estimates for national average emissions from natural gas production, gathering, processing, and transmission within the United States. Although the result is for 2015, satellite assessment of emissions in 2018-202014 found emissions very similar to the 2015 measurement. The result is adjusted downward to remove estimated emissions from oil production and natural gas distribution,15 since those processes are not involved in delivering natural gas for power generation.
We also account for CO2 emissions from production, transmission, and processing of natural gas, which are dominated by emissions from fuel combustion (for compressors) and flaring.
The quantifications discussed above do not include methane and CO2 emissions from LNG liquefaction, handling, and transport, which are potentially large, and these emissions are far less studied, with very few actual measurements, than emissions associated with domestic gas use, so exporting LNG adds significant emissions and uncertainty to overall value-chain emissions. Notably, LNG liquefaction and shipping also emits significant amounts of CO2 from the fuel use involved. We estimate emissions from LNG processes, including CH4 emissions from leaks and uncombusted methane from flares and engines and CO2 emissions from fuel use and flaring, based on mid-range parameters derived from existing literature.16 We note that there have been few measurement studies of methane emissions from liquefaction facilities or LNG tankers, but the expected emissions can be estimated using analogs from oil and gas production processes that have been well measured. We then add these emissions to domestic emissions (production, gathering, processing, and transmission).
Parameters and assumed values are listed in Appendix A. Results broken out by industry segment, for each case discussed below, are listed in Appendix B.
To quantitatively compare lifecycle emissions from generating power with natural gas and coal, we present our estimates of a number of cases involving gas exported as LNG from the U.S. to Vietnam. These are compared to coal exported from Australia to Vietnam.17 In all cases, we compare new power plants to new power plants.18 We use the case of Vietnam as an illustrative example of a rapidly developing country with increasing use of coal for power generation.19 The specifics of the LCA analysis would vary based on specific emissions and operational parameters for other country cases.
Figure 1a. Lifecycle Emissions of Gas and Coal imported to Vietnam for Power Generation (20yr GWP) – national average
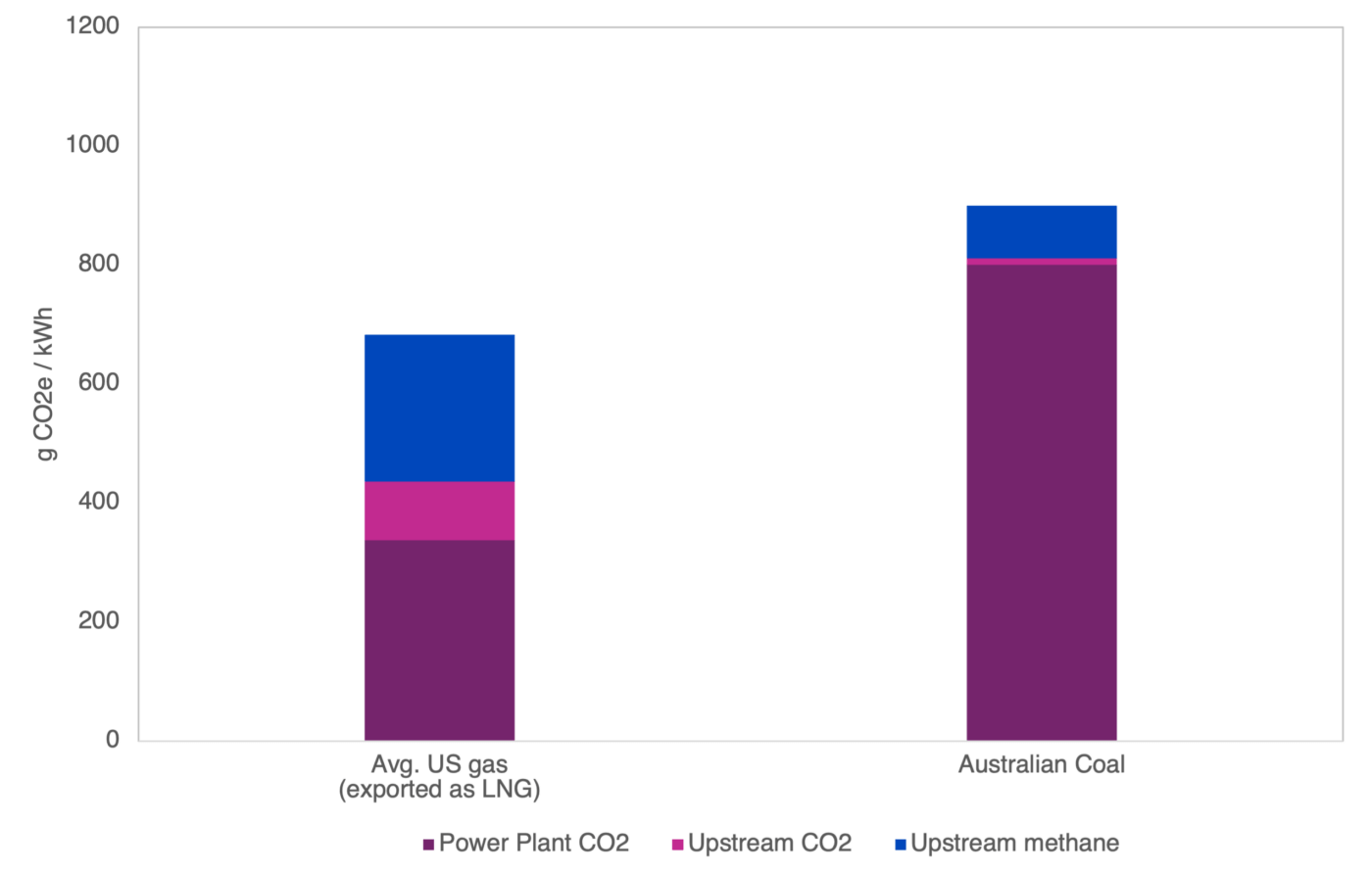
Figure 1b. Lifecycle Emissions of Gas and Coal imported to Vietnam for Power Generation (20yr GWP) – regional cases
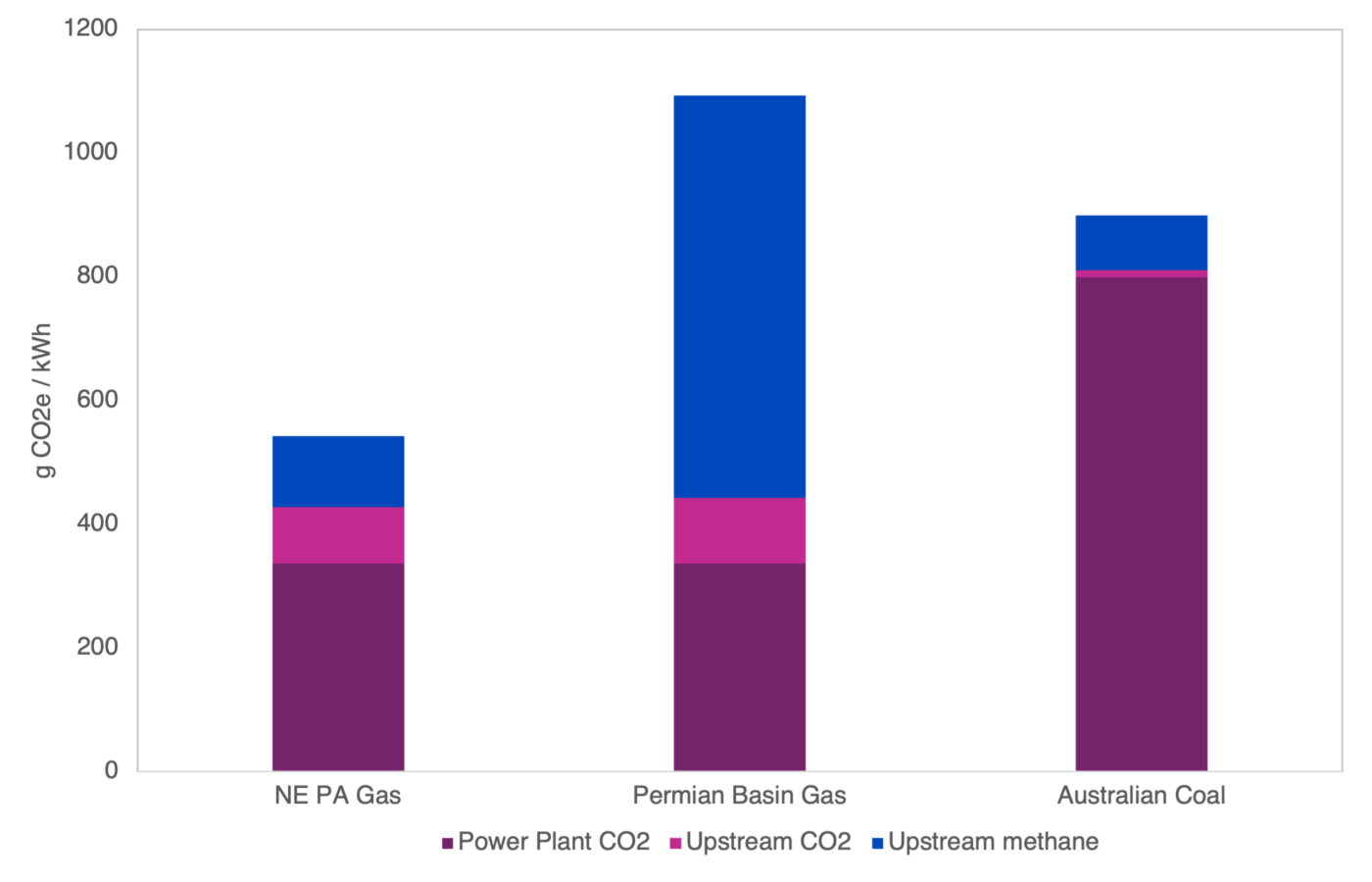
Figure 1a shows our comparison of the lifecycle emissions from using these fuels in Vietnam. The figure shows the amount of greenhouse gas (in CO2e, using 20-year GWPs for methane) emitted per kWh of electricity generated, so it accounts for the greater efficiency of gas-fired generation.
As seen in the figure, the gas-fired power plant emits far less CO2 (58% less) from its stack than the coal-fired power plant. However, when full lifecycle emissions are considered, the relative benefit of gas over coal is much smaller, about 24%. The relative benefit is larger when a 100-year GWP is used, but it is still limited (38%, not shown).
Figure 1a shows our estimate of lifecycle emissions for export via LNG of “average” U.S. natural gas, derived from estimates of nationwide emissions from natural gas systems. However, measurements show that emissions vary significantly among oil and gas producing basins. Figure 1b shows our estimate of lifecycle emissions for exported gas produced in two basins which produce very large amounts of gas, where measured emissions have been at the high and low extremes: gas produced in northeast Pennsylvania, where measured emissions are quite low,20 and gas produced in the Permian basin, where measured emissions are quite high.21
Figure 1b shows that gas in areas such as northeast Pennsylvania can produce significantly less GHG than the U.S. average, but gas produced in the Permian is much worse than the U.S. average. That analysis shows that, depending on upstream methane management practices, imported gas that facilitates coal-to-gas fuel switching could range from reducing receiving country power greenhouse gas impacts by as much as 40% to worsening them by 20%. In addition to this clear difference among basins, even within basins, upstream methane and carbon dioxide emissions can vary significantly.22 U.S. natural gas pipelines are common carriers, and unless there is clear contracting of gas from the source to the point of export, we must be cautious about environmental performance assumptions based only on the basin the gas was produced in.
Finally, we note that the standards which U.S. EPA recently finalized for new sources in the oil and gas sector and the guidelines for existing sources in the sector will substantially reduce methane emissions from some upstream sources. In addition, the European Union recently finalized a methane import standard for imported oil and gas, which could have significant implications for U.S. producers. (The EU is discussing waiving the import standard for countries with equivalent regulations or standards, adding further need for full and proper implementation of the new 111 and Waste Emissions Charge rules.) However, these recently finalized standards do not apply to methane from LNG processes or upstream CO2. Nevertheless, we estimate that with EPA standards and guidelines fully in place, methane emissions upstream of LNG liquefaction will be cut by about 50%. However, as shown in Figure 2, this only reduces the overall LCA of U.S. average gas exported to Vietnam by 13.5%, so this gas would only be ~35% better than “baseline” Australian coal.23
Moreover, it is critical to put this simple coal vs. gas comparison in perspective. For the U.S. average case, gas only reduces emissions by a modest amount – 24% for gas before upstream methane abatement and 35% for gas with upstream methane abatement (using a 20-year GWP). It is particularly important to decarbonize electricity systems rapidly given that decarbonizing other sectors like transportation and buildings will rely heavily on electrification.
Fig. 2. Lifecycle Emissions of Gas and Coal imported to Vietnam for Power Generation (Baseline, with upstream abatement, and with both upstream and CCS on power plant) (20yr GWP)
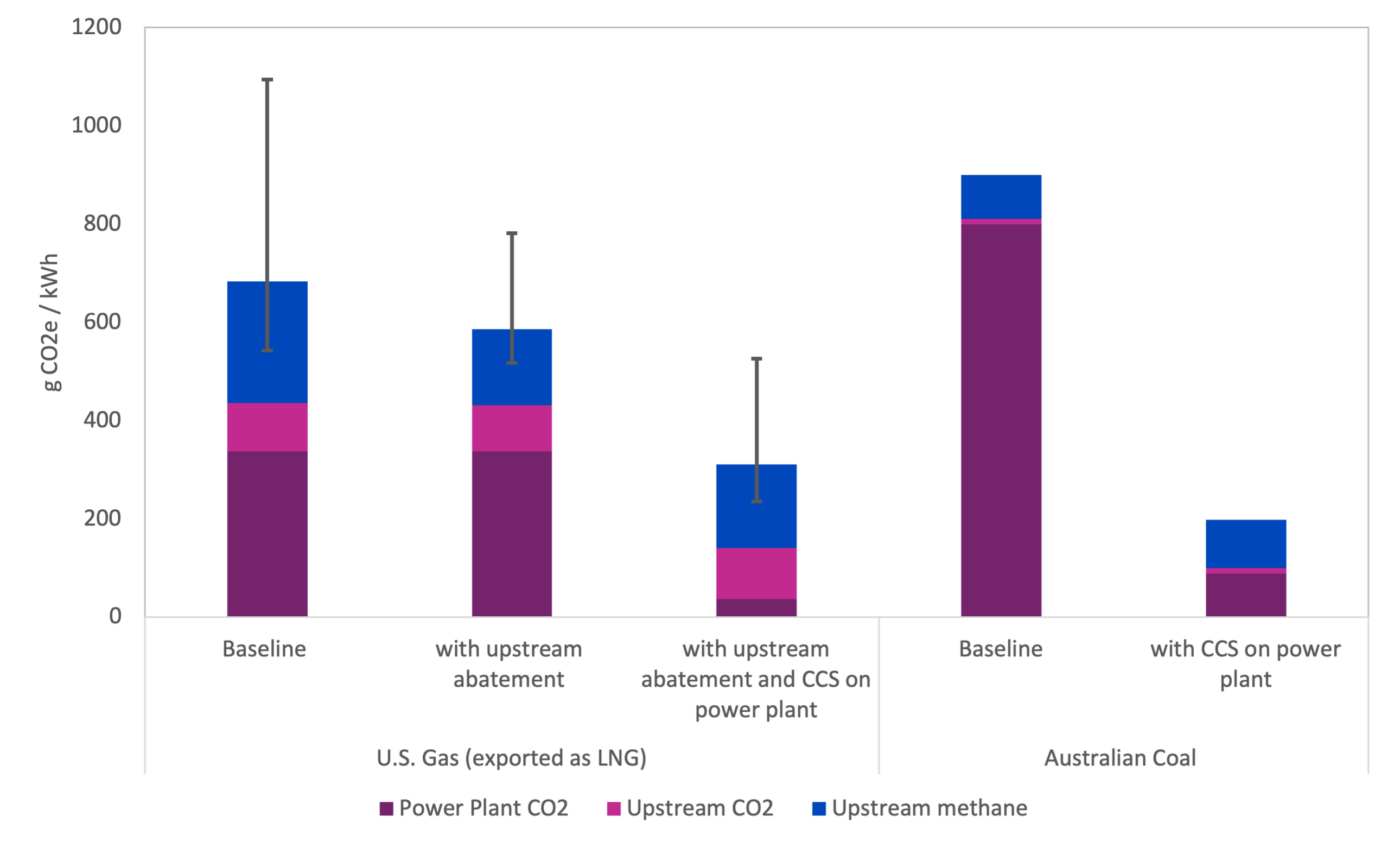
Over the long run, even stringent upstream methane abatement is not sufficient to dramatically reduce the net climate impact of gas-fired power generation towards achieving net zero global emissions. For that, carbon capture and storage would need to be applied to gas fired power generation (as it would for coal generation). The cases “with downstream abatement” in Figure 2 show the greenhouse gas impacts of burning U.S. natural gas, with abatement upstream of the LNG plant consistent with EPA’s rules, and Australian coal to generate power in Vietnam with CCS in place at both power plants (assuming 90% capture of CO2, and a 10% energy penalty for the power plant to operate carbon capture equipment). The impact of both coal and gas generation is considerably lower than the “baseline” cases, but the abated coal case actually shows 37% lower emissions than the abated gas case. This is due to both natural gas’ remaining upstream methane emissions and its upstream CO2 emissions (including substantial emissions from liquefaction and marine shipping).24 While methane management is beginning to be applied throughout the world,25 and carbon capture and storage is beginning to be commercially deployed, significant additional policy and action will be necessary to achieve truly low GHG emissions from gas.
Variation in upstream emissions of natural gas and coal
Upstream CO2 and CH4 emissions from both natural gas and coal vary significantly, from country-to-country, basin-to-basin, and even among companies operating in the same basin. This makes a universal statement about the relative climate impact of natural gas and coal for use in power generation impossible, and it suggests that policy should account for this complexity. As we discuss in the text below, and show in Figure 3, this upstream emission variation is significant.
Above we presented three cases for upstream natural gas with differing leakage rate assumptions: U.S. average (1.8%), northeast Pennsylvania (0.4%), and Permian (6.13%). An analysis of reported CO2 and CH4 in the U.S. demonstrates the variation of emissions intensity among operators working in the same basins.26 Satellite data found a similar variation in emissions intensity among countries, measuring a 2.0% leak rate in the U.S., and among the 20 largest gas producing countries it measured leak rates ranging from 0.2% (Norway) to 7% (Turkmenistan).27
Carbon dioxide emissions associated with producing, processing, and transporting natural gas also vary considerably, reflecting variations in the amount of gas flaring, the quantity of CO2 naturally present in natural gas, and the distances gas travels via pipeline between production regions and export point and via ship as LNG.
Similarly, CH4 emissions from upstream coal production vary significantly, depending on the type of mine; most notably there is a large difference between gassy underground mines and surface mines. In the U.S., based on reported data, average coal emissions are 3.3 kg CH4 / metric ton coal production, with Appalachian underground mining at 5.2 and Powder River Basin surface mining at 0.3.28 And emissions rates similarly vary among countries: a global average of 5.8 kg CH4 / metric ton coal production and a estimates of 0.8, 3.3, and 8.0 kg CH4 / metric ton coal production in Romania, U.S., and China, respectively.29
Fig. 3. Impact of Variable Upstream Methane Emissions on Total Lifecycle Emissions of Coal and Gas (20yr GWP)
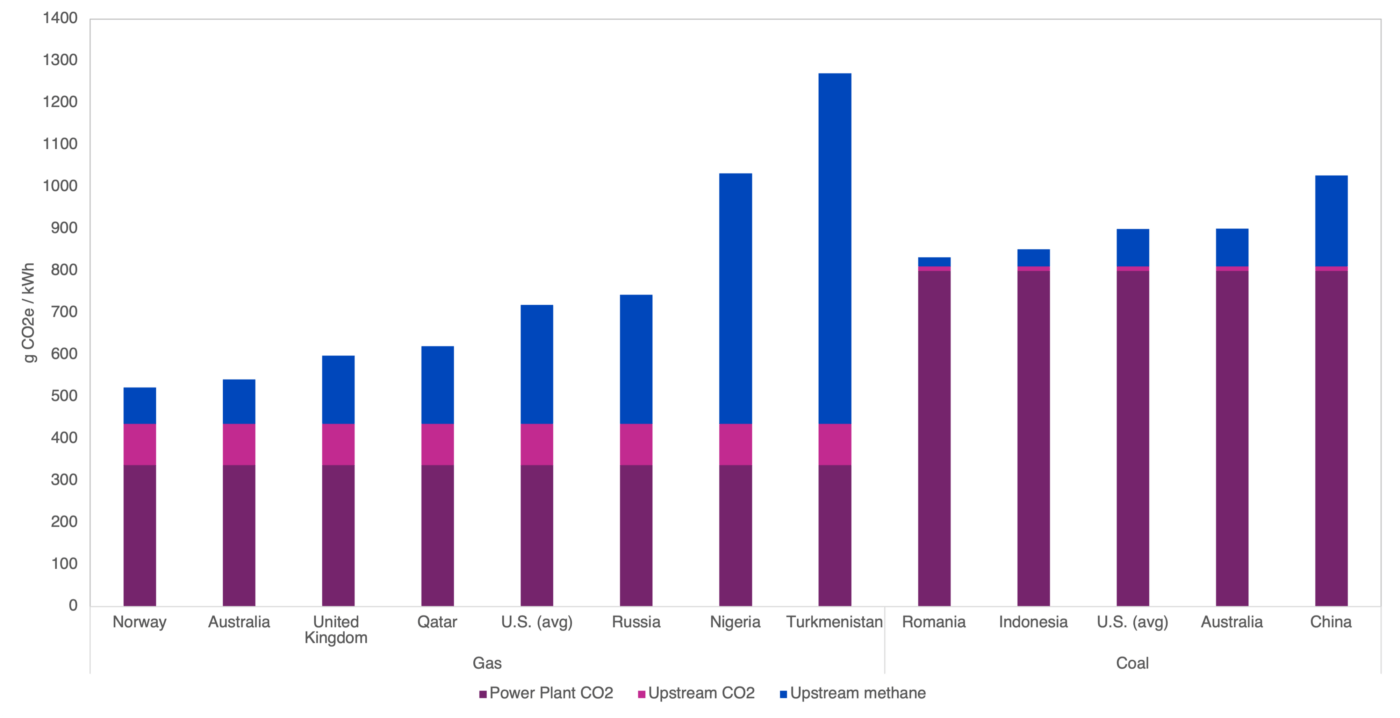
Source: Gas leak rates derived from Shen et al 2023 (except for Qatar which is based on IEA Methane). Coal methane emissions derived from Schwietzke et al 2014a.
Finally, an independent study based on three decades of global atmosphere methane and ethane emissions estimated a global average natural gas leak rate between 2 and 4%, with the balance of the evidence suggesting a global leak rate between 2 and 3%.30 Given the variability of U.S. emissions, and the high emissions from some production basins, available evidence does not show that U.S. gas is cleaner than average global gas, so, even on a simplified basis, displacing other sources of natural gas with U.S. LNG may or may not decrease greenhouse gas emissions, depending on the gas source and upstream emissions mitigation levels.
Mitigation is critical in the short and long term, regardless of LCA analysis
Regardless of the specific lifecycle comparison between natural gas and coal, methane emissions from the natural gas value chain can be substantially reduced (>60%, at least for onshore emissions) in a very short period of time.31 Recently the Biden administration has taken very meaningful steps to reduce methane emissions in the oil and gas sector through the new Clean Air Act Section 111 standards. Congress has also taken important steps to reduce and mitigate methane. It passed legislation that includes measures to reduce methane from the oil and gas sector by establishing the Methane Emissions Reduction Program. But unless these are fully and properly implemented by the States and federal government over the coming years, we won’t see the reductions that are both achievable and necessary. And very importantly, significant methane emissions will remain after these regulations are in place. It is technically possible to further reduce gas industry methane emissions, but new policies will be needed to make this happen.
While the bulk of this analysis focuses on the near term, a critical issue raised by this analysis is whether and under what conditions natural gas has a role in a decarbonized world over the long run. The current analysis shows that the use of natural gas for power generation is only consistent with deep decarbonization if the upstream and downstream CO2 and CH4 emissions from natural gas are maximally mitigated, including methane abatement for gas and utilization of carbon capture and storage for power plant CO2.
Schlussfolgerung
These issues will no doubt continue to be highly polarizing, with some advocating for no expansion of even lower impact fossil fuel use, while many emerging economy leaders insist that global equity requires that they have access to gas and that an immediate transition to all zero-carbon energy is not consistent with human development goals in less wealthy countries. But there also is no doubt that, over the long run, it is essential to aggressively mitigate methane and carbon dioxide emissions from the entire U.S. natural gas supply chain – upstream and eventually downstream all the way to the end use – for the use of exported natural gas in the power sector (or, for that matter, in any other sector) to be consistent with stringent climate goals. Fortunately, we have low-cost options today to greatly reduce the climate impact of whatever gas is used, and there is no excuse for delaying deployment of these options. And we must also work to develop policies that lead to broad adoption of mitigation strategies that go beyond those required under current policy.
Appendix A: Parameters used in lifecycle assessment analysis
Natural Gas Parameters
Case Name | U.S. Average | U.S. Permian (high) | U.S. NE Penn (low) | Einheit |
---|---|---|---|---|
Production Leak Rate as a percent of gas delivered | 1.8 | 6.13 | 0.40 | % |
% of methane from production attributed to natural gas | 60 | 60 | 100 | % |
CO2 emissions per mmcf gas delivered – Combustion/Other | 4.35 | 5.83 | 3.93 | metric tons / mmcf |
CO2 emissions per mmcf gas delivered – Flaring | 0.77 | 1.94 | 0.06 | metric tons / mmcf |
CO2 emissions per mmcf gas delivered – Acid Gas Removal | 0.39 | 0.32 | 0.21 | metric tons / mmcf |
Transmission Pipeline | Yes | Yes | Yes | Yes/No |
Pipeline length for gas transmission in production country | 350 | 772 | 350 | km |
Leak Rate per transmission pipeline km in production country | 0.000004 | 0.000004 | 0.000004 | % per km |
Transportation Leak Rate in production country as a percent of gas delivered | 0.13 | 0.28 | 0.13 | % |
Pipeline length for gas transmission in receiving country | 100 | 100 | 100 | km |
Leak Rate per transmission pipeline mile in receiving country | 0.000004 | 0.000004 | 0.000004 | % per km |
Transportation Leak Rate in receiving country as a percent of gas delivered | 0.04 | 0.04 | 0.04 | % |
Total Upstream Leak rate as a percent of gas delivered | 1.99 | 6.45 | 0.57 | % |
CO2 emissions per transmission pipeline km | .000002 | .000002 | .000002 | Tg per bcf-km |
Heat Rate of Natural Gas Plant | 6,370 | 6,370 | 6,370 | btu / kWh |
Heat content of Natural Gas | 1,037 | 1,037 | 1,037 | btu / cf |
LNG Parameters
Segment | Parameter Name | Low | Med | High | Einheit |
---|---|---|---|---|---|
LNG-Liquefaction | Percent of throughput leaked | 0.01 | 0.04 | 0.07 | % |
LNG-Liquefaction | Percent of throughput consumed as fuel | 5.3 | 10 | 20 | % |
LNG-Liquefaction | Flaring % | 0.008 | 0.409 | 0.8 | % |
LNG-Liquefaction | Un-combusted CH4 (slip) from engine | 0.02 | 0.02 | 0.02 | % |
LNG-Liquefaction | Un-combusted CH4 (slip) from flare | 2 | 5 | 10 | % |
LNG-Shipping | Percent of boil off gas leaked | 2 | 10 | 20 | % |
LNG-Shipping | Speed | 867 | 820 | 772 | km/day |
LNG-Shipping | Round trip distance | km | |||
LNG-Shipping | Tanker Capacity | 260,000 | 215,000 | 175,000 | m3 LNG |
LNG-Shipping | Extra Days for storage at berth and receiving terminal | 0 | 3 | 10 | days |
LNG-Shipping | Boil off/day | 0.1 | 0.125 | 0.179 | % |
LNG-Shipping | Un-combusted CH4 (slip) from engine | 0.1 | 1.7 | 3.13 | % |
LNG-Shipping | CO2 emissions/shipping mile | 407 | 407 | 407 | kg/km |
LNG-Regasification | Percent of throughput leaked | 0.01 | 0.04 | 0.07 | % |
LNG-Regasification | Percent of throughput consumed as fuel | 1.1 | 1.65 | 2.2 | % |
LNG-Regasification | Flaring %-regasification | 0.008 | 0.409 | 0.8 | % |
LNG-Regasification | Un-combusted CH4 (slip) from engine | 0.02 | 0.02 | 0.02 | % |
LNG-Regasification | Un-combusted CH4 (slip) from flare | 2 | 5 | 10 | % |
LNG-Storage Tanks | Percent of throughput leaked | 0.015 | 0.03 | 0.06 | % |
LNG-Ship Loading | Percent of throughput leaked | 0.015 | 0.03 | 0.06 | % |
LNG-Trucking on receiving side | Percent of throughput leaked | 0.015 | 0.03 | 0.06 | % |
Coal Parameters
Case Name | Australia Coal Case New | China Coal Case New | Australia Coal Case Old | China Coal Case Old |
---|---|---|---|---|
CH4 per metric ton for coal production | 3.3 | 8.0 | 3.3 | 8.0 |
CO2 per metric ton for coal production | 5,489.0 | 5,489.0 | 5,489.0 | 5,489.0 |
Coal Rail Transport | Yes | Yes | Yes | Yes |
CO2 per metric ton for coal transport per mile (rail) | 11.3 | 11.3 | 11.3 | 11.3 |
Miles coal rail/truck/barge transport | 260.0 | 500.0 | 260.0 | 500.0 |
CO2 per metric ton for coal transport (ocean), import/export terminals | 1,474.0 | 1,474.0 | 1,474.0 | 1,474.0 |
CO2 per metric ton for coal transport per mile (ocean) | 6.3 | 6.3 | 6.3 | 6.3 |
Miles coal ocean transport | 3,900 | - | 3,900 | - |
Heat Rate of Coal Plant | 8,369.0 | 8,369.0 | 10,655 | 10,655 |
Heat content of coal | 25.50 | 25.50 | 25.50 | 25.50 |
Appendix B: Results for cases presented in figures
Table B1. Results for cases presented in text/figures, using 20-year GWP for methane
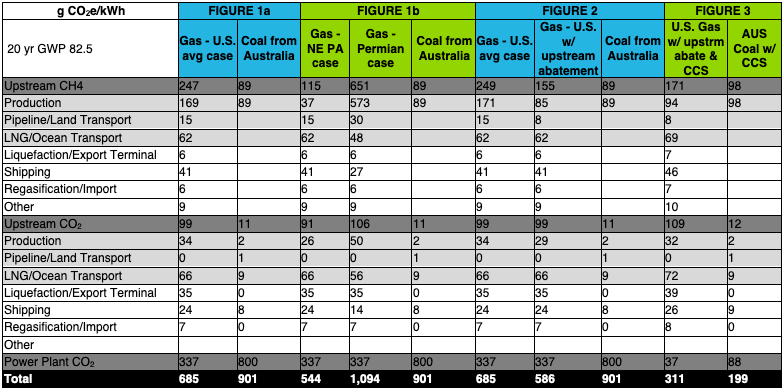
Table B2. Results for cases presented in text/figures, using 100-year GWP for methane
Note: These results are not presented in the figures, which show results from Table B1
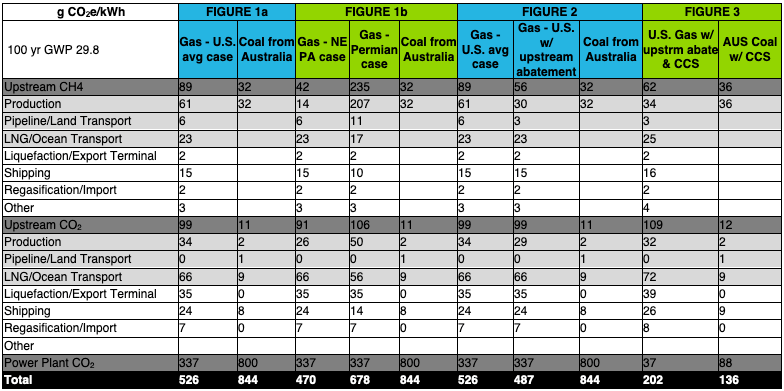
Fußnoten
- Globally only 40 percent of natural gas is used for electricity production (International Energy Agency, Gas Market Report, Q3-2022, July 2022, p. 26.). This analysis below does not apply to other uses of exported natural gas.
- Paul, Corey, “LNG Project Tracker: Contracting surge accelerates next cycle of export projects” July 14, 2022.
- IEEFA “Global LNG Outlook 2023-27”.
- Yang et al 2022 “Pipeline Availability Limits on the Feasibility of Global Coal-to-Gas Switching in the Power Sector”.
- Smillie et al 2022 “Greenhouse Gas Estimates of LNG Exports Must Include Global Market Effects”, McJeon et al 2014 “Limited impact on decadal-scale climate change from increased use of natural gas”, Greiner et al 2018 “Snakes in The Greenhouse: Does increased natural gas use reduce carbon dioxide emissions from coal consumption?”.
- McJeon et al. 2014.
- Gilbert and Sovacool 2017 “U.S. liquefied natural gas (LNG) exports: Boom or bust for the global climate?”.
- Smillie et al. 2022.
- Kemfert et al. 2022 “The expansion of natural gas infrastructure puts energy transitions at risk”.
- In this paper we use the term “upstream” for all processes / emissions that occur upstream of the power plant (before the power plant). This is different from some usage of the term “upstream” in natural gas systems, which can be limited to gas production, or gas production and gathering, boosting, and occasionally processing.
- See IPCC’s Sixth Assessment Review, Working Group I Report, Chapter 7, Table 7.15 (the appropriate GWPs for methane emissions from natural gas systems are “CH4-fossil”).
- See for example Brandt et al 2014 “Methane Leaks from North American Natural Gas Systems”, Alvarez et al 2018 “Assessment of methane emissions from the U.S. oil and gas supply chain”, and references therein.
- Alvarez et al 2018.
- Shen et al. 2022 “Satellite quantification of oil and natural gas methane emissions in the U.S. and Canada including contributions from individual basins”.
- Alvarez et al. estimates methane emissions for oil and natural gas production combined. We assume that 60% of production emissions is attributable to gas, based on gas’s portion of energy production from those sites.
- See e.g. Balcombe et al. 2022, Mallapragada et al. 2018, Safaei et al. 2015, Lowell et al. 2013, Carbon Limits 2021, Kwak et al. 2018, Abrahams et al. 2015, DOE/NETL 2021, and Johnson 2018.
- Methane emissions for Australian coal: Schwietzke et al. 2014. CO2 for coal mining/transport: Skone et al. 2016.
- Efficiencies: New NGCC heat rate: 6,370 btu / kWh (54%), New coal plant heat rate: 8,369 btu / kWh (41%).
- Global Energy Monitor. “Newly Operating Coal Plants by Year, 2000-2023 (MW)”.
- Barkley et al 2017 “Quantifying methane emissions from natural gas production in north-eastern Pennsylvania”.
- Sherwin et al. 2023 “Quantifying oil and natural gas system emissions using one million aerial site measurements”, Lyon et al. 2021 “Concurrent variation in oil and gas methane emissions and oil price during the COVID-19 pandemic”, Shen et al. 2022.
- Benchmarking Methane and Other GHG Emissions of Oil & Natural Gas Production in the United States, 2023.
- See Appendix B.
- See Appendix B.
- E.g. A Methane Champion: Colombia becomes first South American country to regulate methane from oil and gas, EU agrees to regulation that will dramatically cut EU and global methane pollution, Canada reaffirms commitment to curb methane emissions with proposed oil and gas standards, Mastering methane: How Nigeria and Clean Air Task Force are taking on methane pollution together.
- Benchmarking Methane and Other GHG Emissions of Oil & Natural Gas Production in the United States, 2023.
- Shen et al 2023.
- Environmental Protection Agency (EPA) “Inventory of U.S. Greenhouse Gas Emissions and Sinks.”
- Schwietzke et al 2014a “Global Bottom-Up Fossil Fuel Fugitive Methane and Ethane Emissions Inventory for Atmospheric Modeling”.
- Schwietzke et al 2014b “Natural Gas Fugitive Emissions Rates Constrained by Global Atmospheric Methane and Ethane”.
- CATF Reducing Methane from Oil and Gas: A Path to a 65% Reduction in Sector Emissions, IEA Methane Tracker 2023.