Carbon Capture and Storage in Europe FAQs
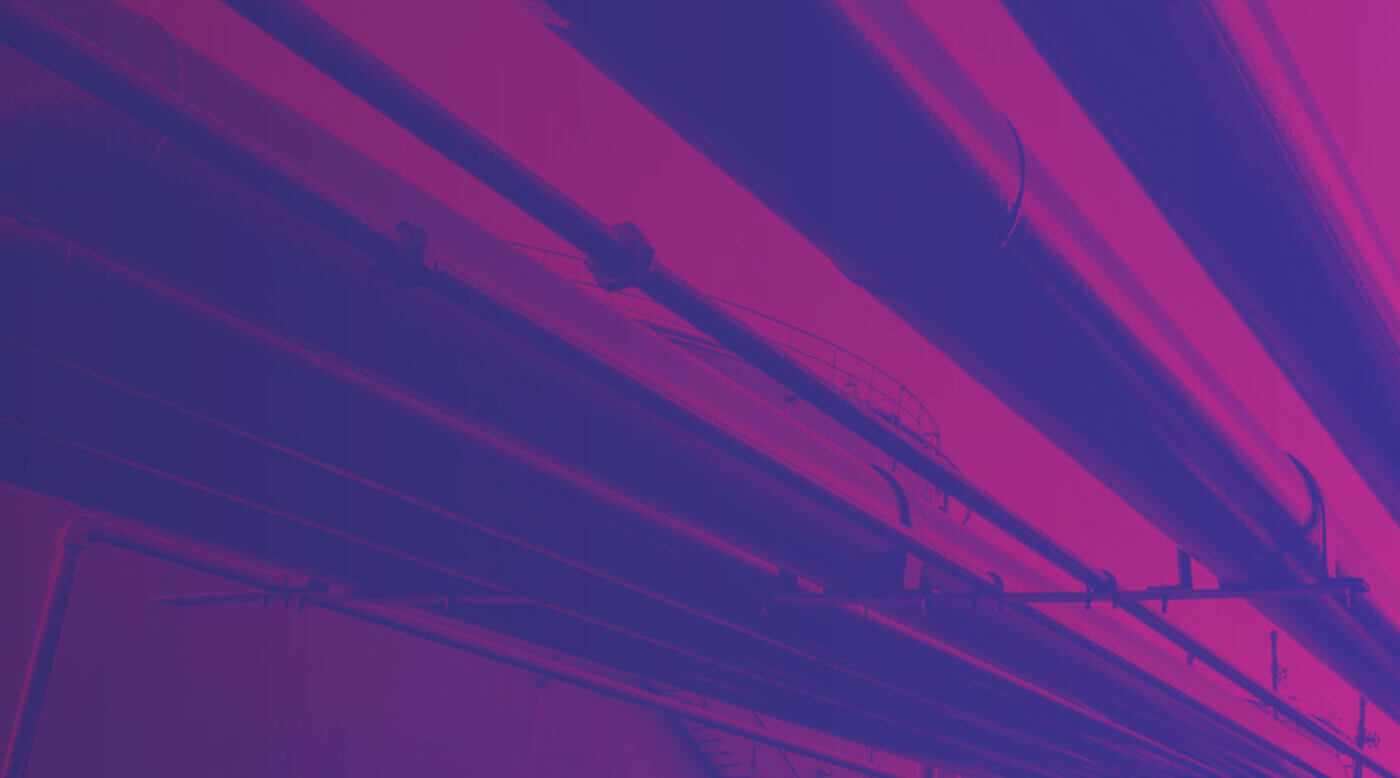
- What is carbon capture and storage and why does Europe need it?
- CO₂ storage
- CO₂ capture
- How can carbon capture and storage be deployed in Europe?
- What are the risks associated with carbon capture and storage?
- Are there alternatives to carbon capture and storage?
- Using CO₂ storage for carbon dioxide removal
What is carbon capture and storage and why does Europe need it?
What is carbon capture and storage?
CO₂ can be permanently prevented from entering the atmosphere and driving climate change by injecting it into certain types of rock formation found deep underground. The CO₂ must first be ‘captured’ by separating it from the CO₂-rich exhaust gases of industrial plants. The CO₂ capture process can use many different technologies, but currently the most frequently used solutions employ chemicals that selectively bind CO₂ and then release the gas when heated. CO₂ often needs to be transported to the storage location, which can be by pipeline, ship, rail, or road.
Figure 1: A simplified illustration of carbon capture (left) and storage (right)
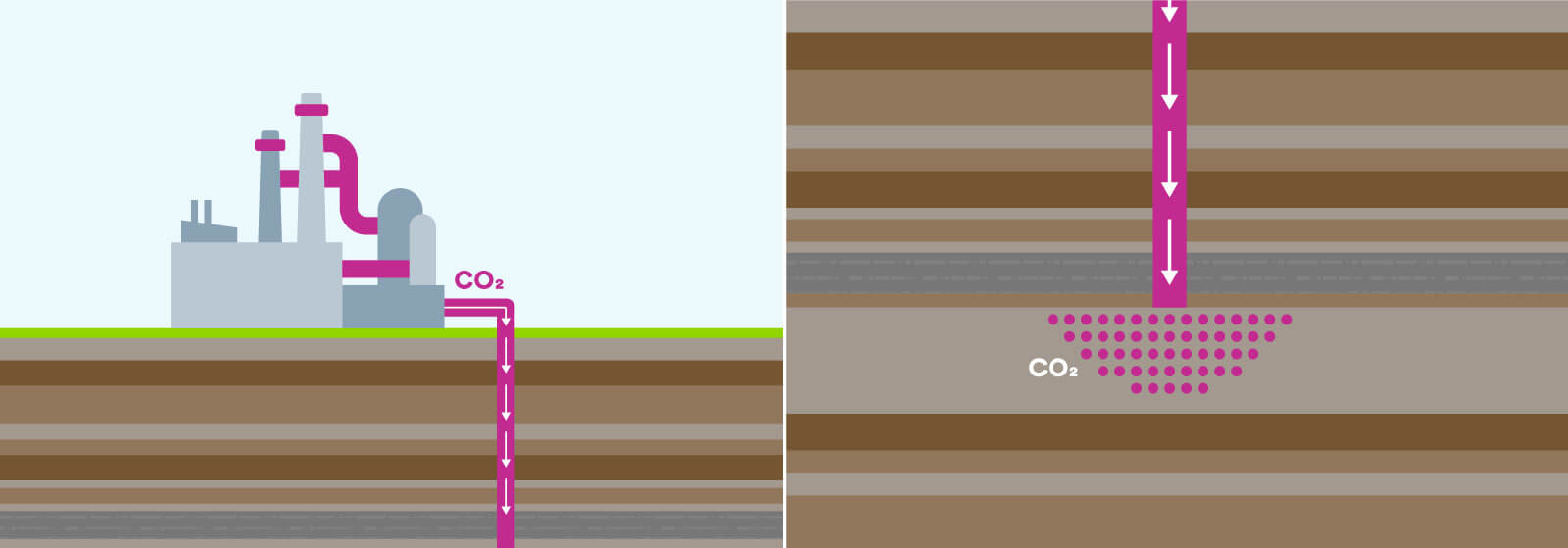
Why does Europe need carbon capture and storage to meet its climate goals?
Carbon capture is most needed to deal with CO₂ emissions from industrial sectors with few or no other options available, either because they inherently release CO₂ from a chemical process, or because they are very difficult to power with renewable electricity alone. This includes emissions from cement, chemicals such as fertilisers, waste incineration plants, and steel plants.
Permanent removal of CO₂ from the atmosphere will also be needed to balance any remaining emissions from sectors that are even more costly to decarbonise, such as aviation, and to reverse any ‘overshoot’ of acceptable atmospheric levels of CO₂.
The European Climate Law requires the EU to reach net zero greenhouse gas emissions by 2050 – a goal which is consistent with the International Panel on Climate Change’s (IPCC) recommendation to limit global warming to 1.5°C. Studies of how Europe can reach net zero in time nearly all show a significant role for carbon capture and storage technologies, both to cut CO₂ emissions from industry and remove CO₂ from the atmosphere. These include analysis by the IPCC, the International Energy Agency, and the European Scientific Advisory Board on Climate Change (Figure 2). The EU’s own analysis has estimated around 300 to 600 million tonnes a year of CO₂ may need to be captured by 2050 – around 11 to 22% of the EU’s CO₂ emissions in 2021.
Figure 2: Estimates of the quantities of CO₂ which would be captured in Europe to reach net zero in 2050, according to various studies by the European Commission, DNV, the IPCC, the IEA and the European Scientific Advisory Board on Climate Change (EU only)
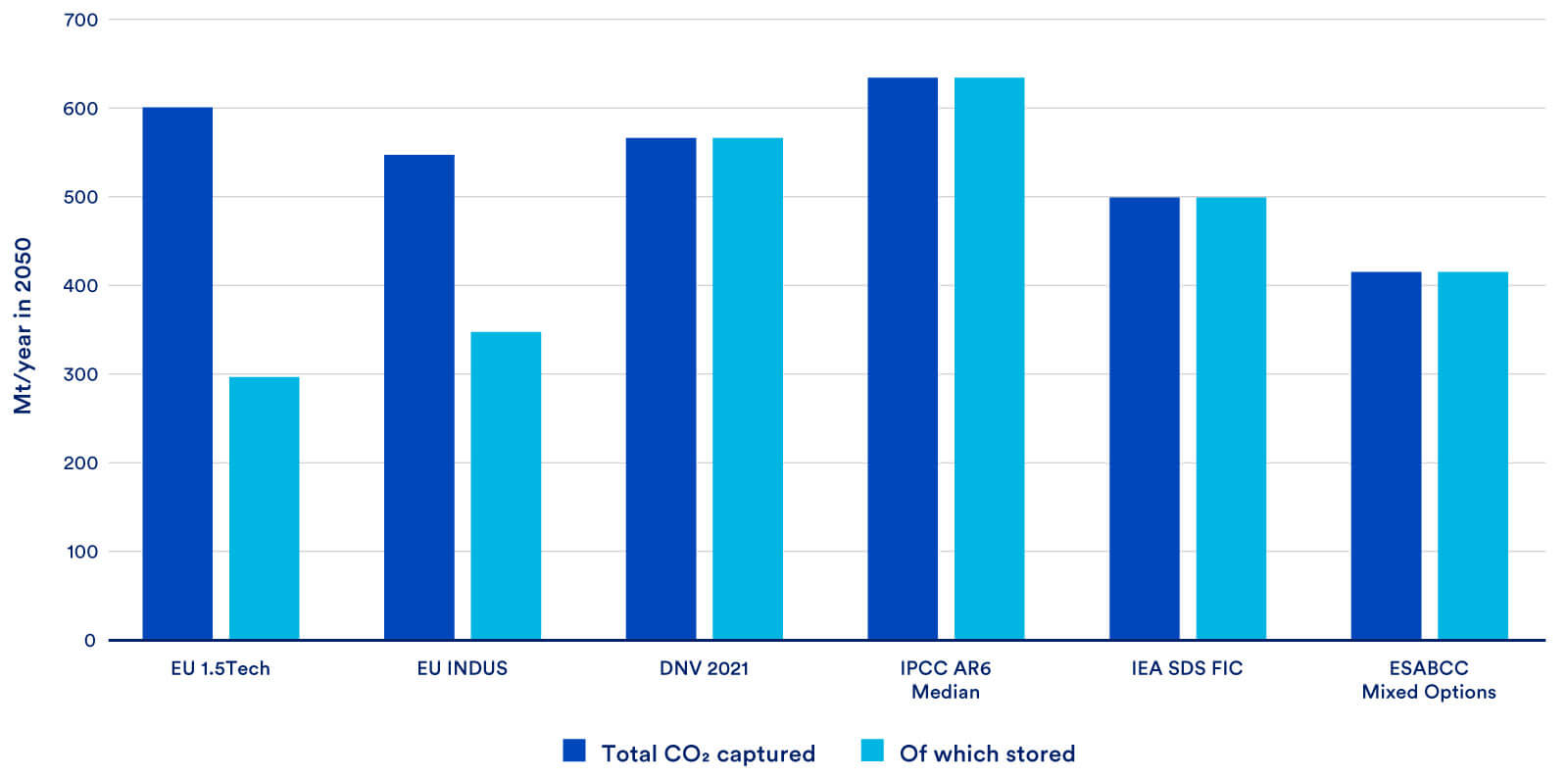
While we can’t know exactly how much carbon capture and storage will be required, it is clear that this technology will need to be scaled up very rapidly. Decarbonising Europe in less than 30 years is an unprecedented challenge that will require a wide range of technologies to tackle different parts of the economy and different countries. Having more technologies available also reduces the climate risk of other solutions failing to develop fast enough.
Is the technology proven at full scale?
The complete process of capturing and storing manmade CO₂ is demonstrated today at over 40 commercial sites around the world, with the earliest starting in 1972. Many of these plants are in North America, where CO₂ is widely used to increase oil production but becomes stored underground as a result. Five operating facilities store CO₂ in deep ‘saline reservoirs’ – purely for climate benefit – including the Sleipner and Snøhvit projects in Norway. Sleipner has been separating CO₂ from natural gas and safely storing it beneath the North Sea since 1996.
However, the individual technologies involved in carbon capture and storage are much more widespread:
- CO₂ separation technology has been used for nearly 100 years to remove CO₂ from natural gas.
- Some other industries, such as fertiliser and bioethanol production, produce relatively pure CO₂ or already carry out CO₂ separation as part of the process.
- Over 160 million tonnes of CO₂ are captured annually for use in industries such as food and drink or fertiliser production.
- There are over 8000 km of pipelines carrying CO₂ in the USA and 300 km of CO₂ pipeline in the Netherlands.
Existing carbon capture technologies now need to be used at large scales in a wider range of polluting facilities. The technologies used to capture CO₂ can differ according to the industry where they are applied, but typically use very similar processes to those used commercially today. Carbon capture has been successfully trialled at large scales for many new applications, and full-scale versions for some key sectors – including cement and waste incineration – are currently under construction in Europe.
CO₂ storage
How is CO₂ stored in geological formations?
CO₂ storage takes place at depths of more than 800 metres underground, where the high pressures ensure that CO₂ remains in a liquid-like state, trapped within the tiny pores found in rocks such as sandstone (Figure 3). These rock formations can be spent oil and gas reservoirs or contain very salty water – these are known as saline aquifers or saline reservoirs.
Suitable storage sites are carefully chosen to have a denser, impermeable layer of ‘cap rock’ lying above the porous rock, which prevents CO₂ from moving upwards towards the surface. Over time, the CO₂ becomes increasingly securely bound as it dissolves in water and can eventually – over hundreds of years – turn into solid minerals (Figure 4). The European Union (EU), Norway, and the UK have comprehensive legal frameworks to regulate CO₂ storage activity. These require new storage sites to be thoroughly assessed for suitability before a permit to store CO₂ is granted, as well as ensuring that operators constantly monitor the store for leakage and demonstrate the CO₂ is stable before closing the site.
Figure 3: CO₂ storage
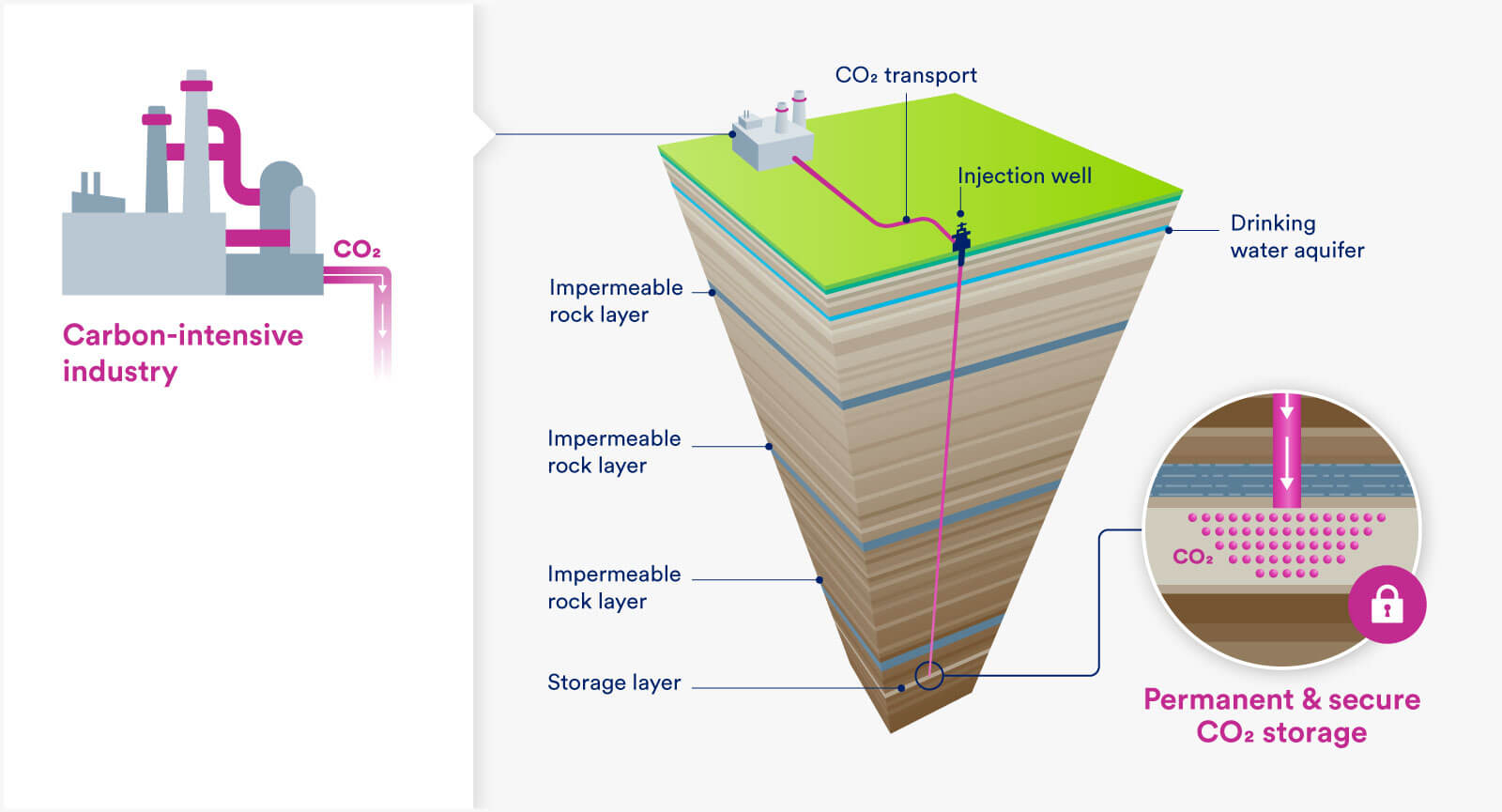
Figure 4: How CO₂ becomes bound (‘trapped’) by various mechanisms within the storage reservoir
CO₂ is initially prevented from moving upwards by impermeable caprock (structural trapping). It also becomes held within rock pores by capillary forces (residual trapping) and dissolves in brine over time (solubility trapping). Eventually, a portion of the dissolved CO₂ reacts with the rock to form solid mineral carbonates (mineral trapping). Aside from structural trapping, all these forms of trapped CO₂ would require deliberate human intervention to reverse.
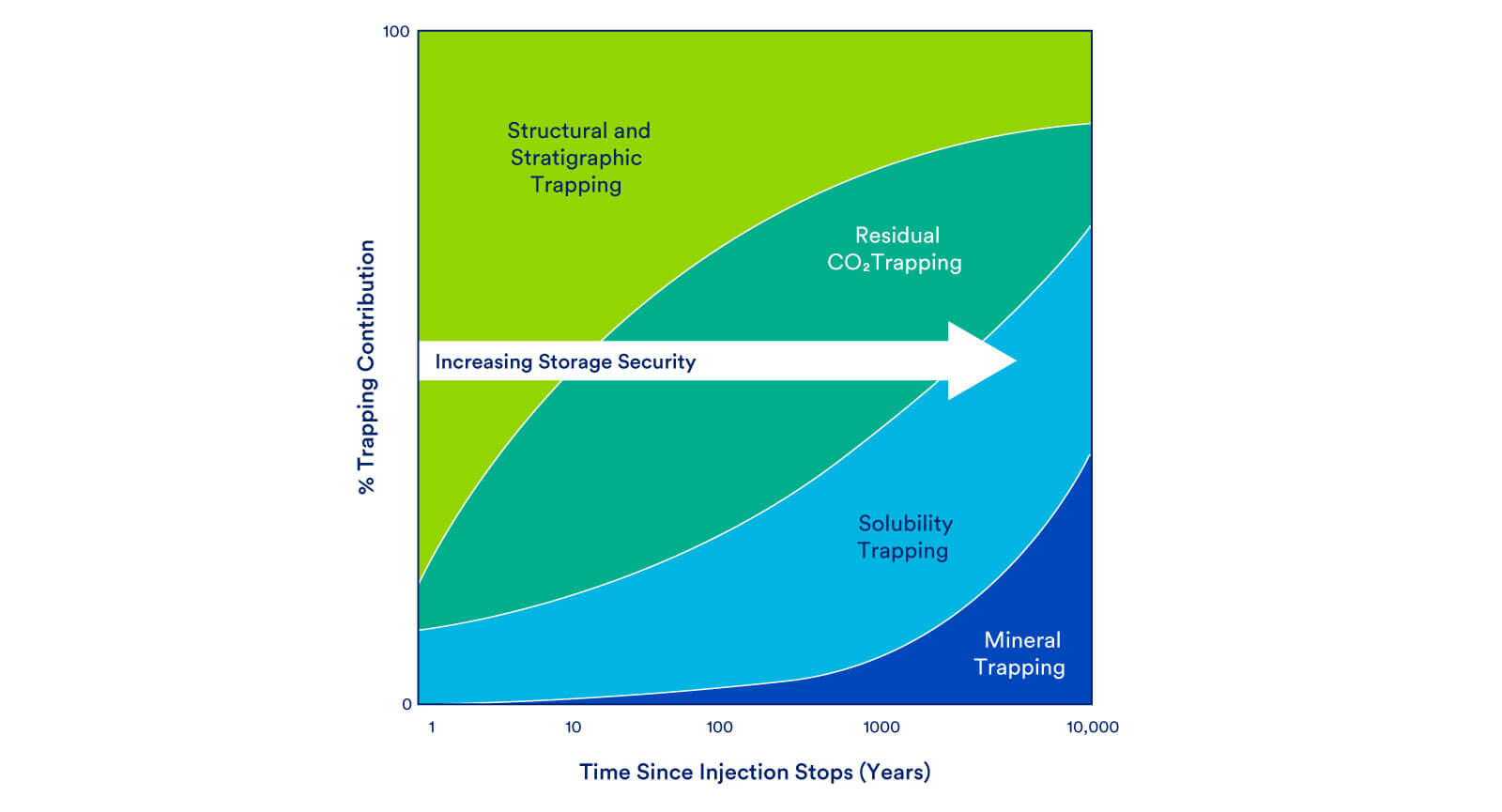
How do we know CO₂ will be stored permanently?
CO₂ leakage from properly assessed and characterised storage sites is considered extremely unlikely. Similar geological structures have kept oil and gas, and even many natural stores of CO₂, trapped in place for millions of years. The International Panel on Climate Change has stated that CO₂ stored in geologic formations is likely to remain ‘permanently isolated from the atmosphere’ or ‘for more than a 1000 years’ if the storage site is appropriately selected. No manmade sites developed for the purpose of CO₂ storage have ever been known to leak.
Under European legislation, storage sites must be thoroughly assessed for their potential to permanently store CO₂, including computer modelling of how the stored CO₂ will behave over centuries. Operational storage projects then use sensitive monitoring techniques that can track the underground spread of CO₂ (Figure 5). This takes place during injection and long after injection stops to verify that the CO₂ remains in place and has reached a stable state.
As shown in Figure 4, a growing proportion of CO₂ becomes bound within the rock, and would need human intervention to be released. Possible leakage pathways for the remaining ‘free’ CO₂ include abandoned manmade wells or, to a lesser extent, through natural geological faults. Regulations in Europe require any wells in the area to be identified and sealed before CO₂ is injected.
If leakage of stored CO₂ were to occur, it would likely be at rates that are so low the CO₂ storage would still have significant value as a climate solution. Acceptable leak rates are generally determined to be less than 0.01% per year from the store, which would retain 99% of the CO₂ over 100 years. A study of potential leak global leak rates, based partly on leakage from gas reservoirs and natural CO₂ stores, projected average annual leakage rates of up to 0.0002% in a well-regulated industry. Similarly, a study of CO₂ storage permanence for the UK government estimated that a worst-case scenario might amount to around 0.07% of the stored CO₂ leaking over a 100-year period.
Figure 5: Visualisation of CO₂ stored by the Sleipner project using seismic imaging, with bright areas showing the presence of CO₂ in rock layers
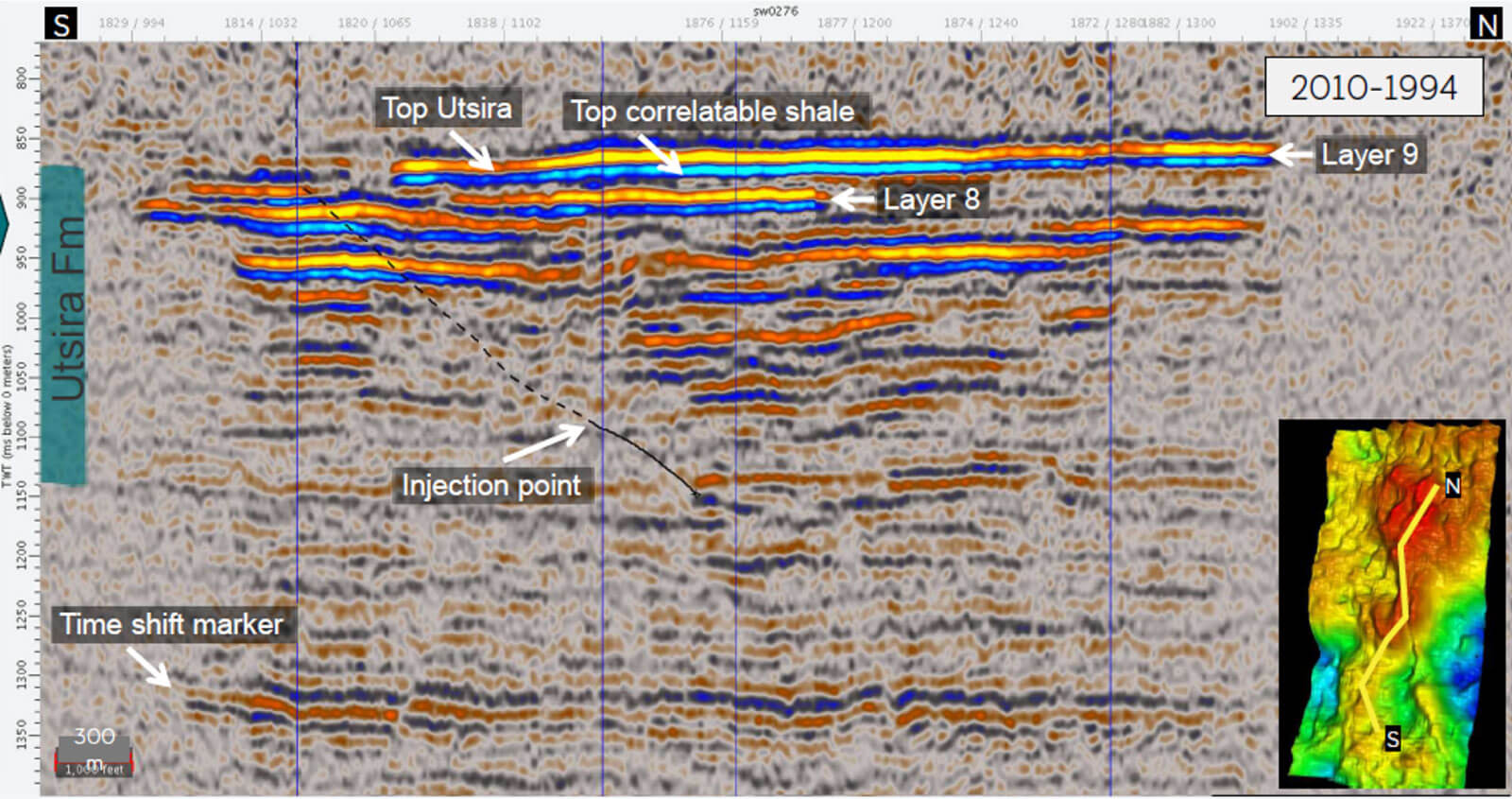
Is there enough space to store the CO₂?
Initial assessments have indicated that Europe has the capacity to store up to 500 billion tonnes of CO₂, or around three hundred years’ worth of CO₂ emissions from all the region’s industry and power generation. CATF analysis highlights that large areas of the region have suitable geology for CO₂ storage, but seismic mapping and exploratory wells are needed to better characterise individual locations and more accurately determine the available capacity. Currently, detailed assessments of potential CO₂ storage sites are often limited to areas that have already been explored for oil and gas.
Is carbon capture and storage used to produce more oil?
Most of the CO₂ stored today is injected for the purpose of ‘enhanced oil recovery’ in North America, where CO₂ is pumped underground to increase oil production from older wells. This is because, until recently, there has been no other incentive for storing CO₂ underground. While CO₂ can be measurably and permanently stored during enhanced oil recovery, climate policies are helping to ensure that new CO₂ storage plans are largely focused on storage for its own sake – without fossil fuel production.
In Europe, there are no plans for CO₂ storage with enhanced oil recovery and it is explicitly excluded from contributing to the European Commission’s proposed CO₂ storage target. Several of the proposed CO₂ storage sites in Europe use old oil and gas reservoirs, as these often offer a lower-cost option for early developments. However, their use as storage sites requires a change in operating licence that is very unlikely to be reversed to continue production.
CO₂ capture
How does CO₂ capture work?
CO₂ needs to be in a relatively pure state before it can be safely transported and stored underground. Other gases and impurities can interfere with these processes or increase the energy required to compress the CO₂. However, different kinds of industries produce CO₂ at a wide range of concentrations, from as low as 4% (from burning natural gas) to 95% (ammonia production). This is why most polluting facilities need to use technologies that separate out the CO₂ from the other gases they produce. The leftover gases often consist largely of nitrogen from the air.
For dilute CO₂ (below 20%), the most commonly used technologies are solutions of organic chemicals called alkanolamines – or simply ‘amines’ – which readily bind to CO₂ but are able to release it when heated. Using amines for CO₂ separation was first patented in the 1920s and has been widely used since then to remove CO₂ from natural gas, as well as to provide CO₂ for commercial uses. The gas mixture passes upwards through tall towers packed with material, while the amine solution flows downwards and reacts with CO₂. Now rich in CO₂, the solution is sent to another reactor where it is heated to release the pure gas (Figure 6).
Figure 6: A CO₂ capture process using amine absorption
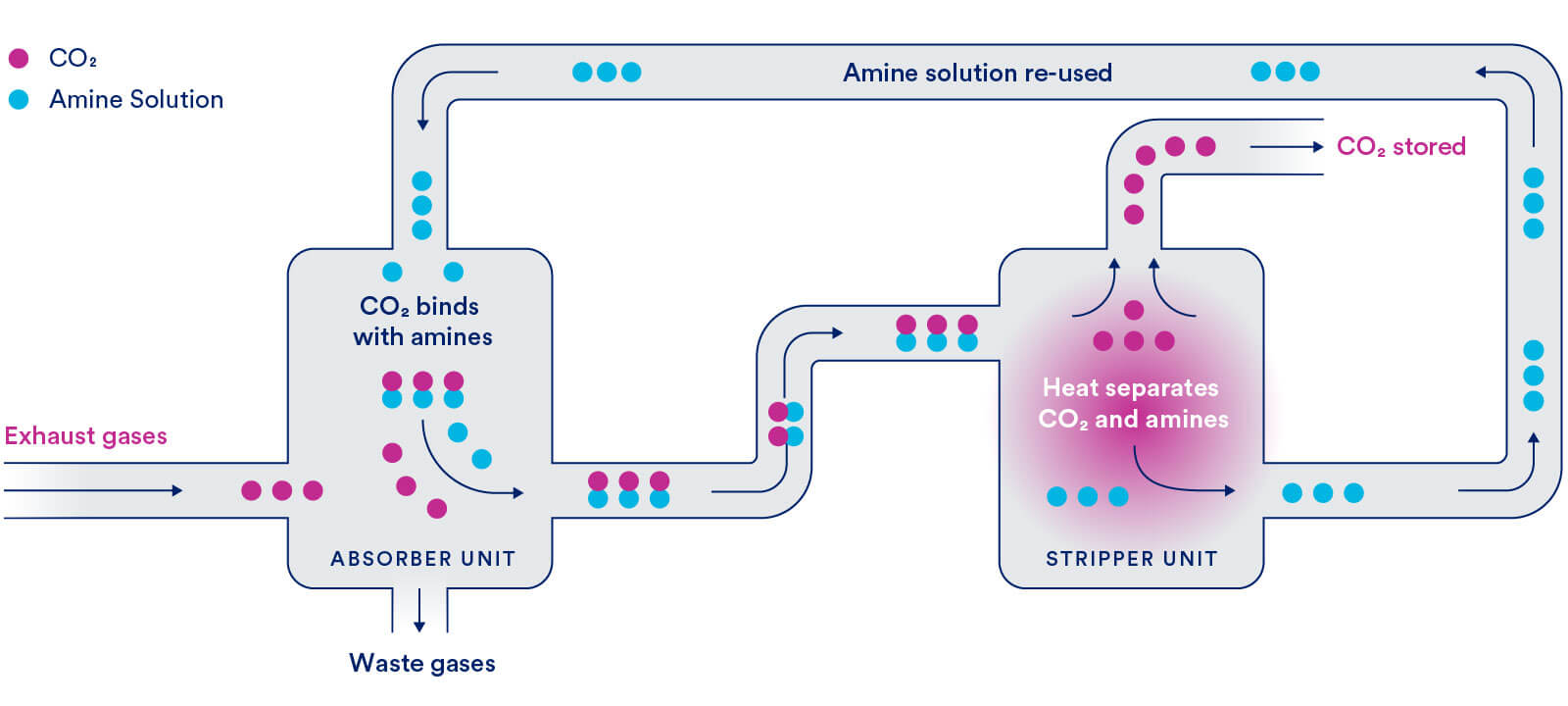
There are many alternative technologies for separating CO₂ used commercially today, particularly for gas mixtures with higher CO₂ concentrations or at higher pressures. These include thin membranes which only allow CO₂ to pass through, or solid materials which selectively bind CO₂. Cooling the gas mixture down to very low temperatures can also allow CO₂ to be removed as a liquid or solid.
We have the technologies available to capture high purity CO₂ from any industrial exhaust gases, but different technologies will be best suited to different applications, and improvements continue to be made. There is considerable research interest in developing new technologies or optimising existing ones to use less energy, cost less, or have other environmental benefits.
Is carbon capture energy intensive?
Like most pollutant control technologies, carbon capture consumes energy – first to separate CO₂ from other gases and then to compress it for transportation and storage. The amount of energy needed for the separation depends on what kind of industrial process is producing the CO₂ and which technology is used to carry out the separation.
Some industries, such as oil refineries or fertiliser plants, produce quite concentrated CO₂ streams which can be separated with relatively little energy penalty. Others, including cement or waste-to-energy plants, have more dilute streams which are more challenging. For these sources, today’s most mature capture technologies generally consume at least 2 gigajoules of heat energy per tonne of CO₂ captured. If this energy were supplied by natural gas combustion, roughly another 120 kg of CO₂ would be produced for every tonne captured, and these emissions would also need to be captured.
However, the energy required for carbon capture does not have to come from additional fossil fuel consumption. Many industrial producers produce waste heat that could be used to drive some CO₂ capture processes, while some technologies can be driven by green electricity. The carbon capture process itself also produces waste heat that some projects plan to reuse, for instance, in residential heating – effectively offsetting some of the energy penalty.
Can carbon capture prevent all the CO₂ emissions from an industrial facility?
Many operational and planned CO₂ capture projects are designed to capture around 90% of the emissions from an individual facility. Older projects that have not been designed with climate benefit in mind (but used for oil production) may capture even less. However, even with today’s technologies there is no technical barrier to capturing up to 100% of the fossil CO₂ from many industries, and several new projects are now aiming for levels of at least 95%. Capturing more CO₂ carries a higher cost, but studies have shown that the increase can be relatively small compared to the whole project cost (Figure 7).
The proportion of CO₂ captured by some operating projects can also look smaller where only one CO₂ source within a larger plant has been treated, or if only a portion of the CO₂ stream has been treated – usually in order to trial a new technology. This does not mean that it not possible to use the same technology to capture the rest of the emissions, only that the right incentives have not yet been in place.
Figure 7: How the cost of capturing CO₂ increases with increasing capture rate for gas (NGCC) and coal (PC) power plants
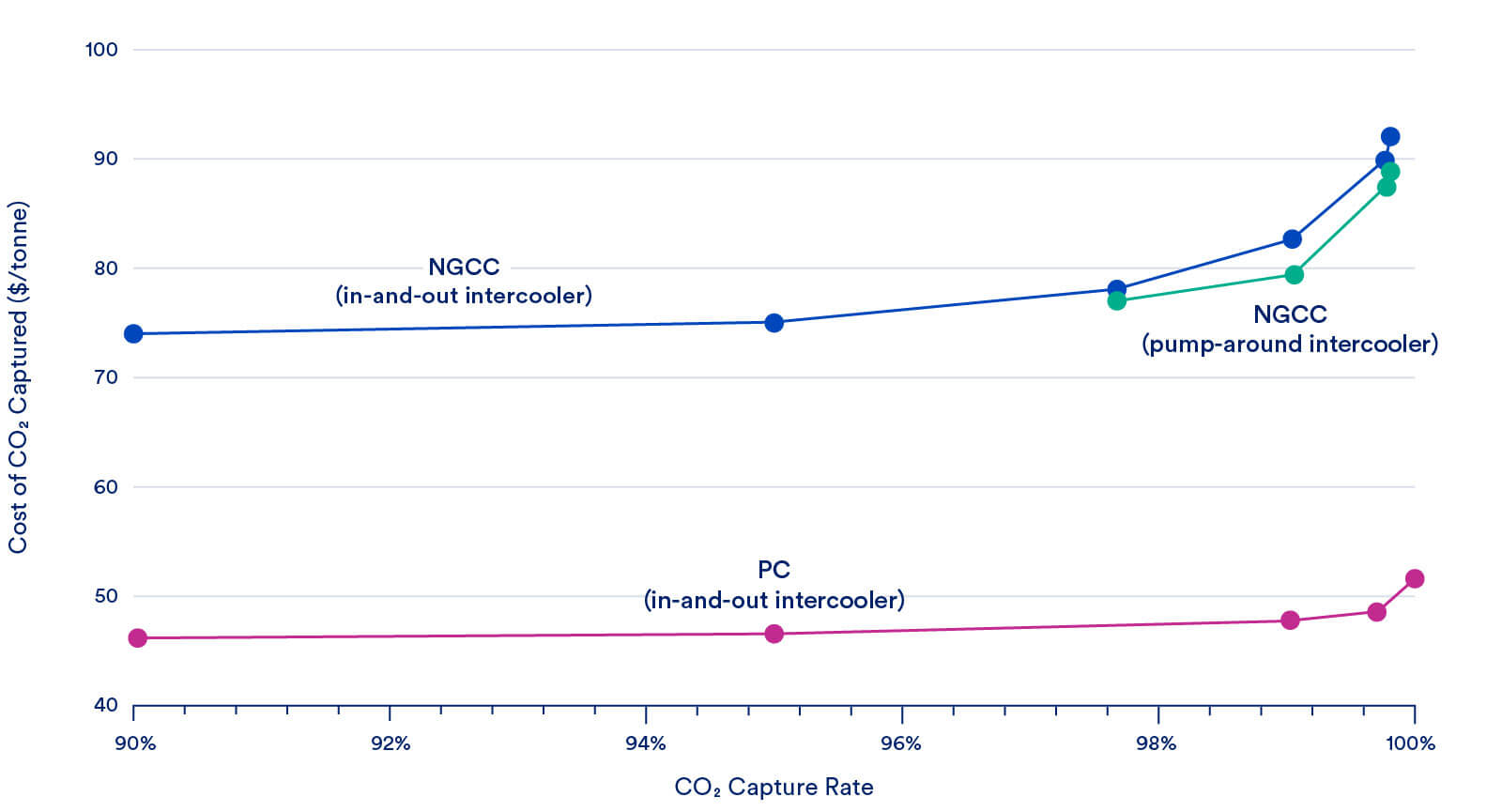
How can carbon capture and storage be deployed in Europe?
Where is carbon capture and storage already being developed in Europe?
The storage of CO₂ beneath the Norwegian North Sea has been safely demonstrated since 1996. In the last two years, the urgency of the net zero goal and growing awareness of the need for carbon capture and storage has led to growing political support for the technology in Europe and many other countries. CATF’s project map identifies around 100 carbon capture or storage projects proposed in Europe today; many of these are located around the North Sea, where there is well-understood geology due to years of exploitation of oil and gas.
In 2020, Norway provided significant funding towards the development of a new storage site in the North Sea, together with CO₂ capture from a cement plant and a waste-to-energy plant in Oslo. These projects are currently construction and expected to start in 2025 (Figure 8). The Netherlands has included carbon capture and storage in its subsidy scheme for low-carbon technologies, enabling an initiative to capture and store 2.5 million tonnes per year of CO₂ from various facilities in Rotterdam; this is expected to become operational by 2026. The EU has selected 13 large-scale carbon capture and storage projects to receive financial support through its Innovation Fund for decarbonising technologies, located in France, Finland, Sweden, Belgium, Iceland, Bulgaria, Greece, Croatia, Germany and Poland. The UK has set a target of capturing and storing up to 30 million tonnes of CO₂ by 2030, and is aiming to have at least two major projects operational by 2026. Denmark, Sweden, France, and Germany are all also implementing national policies to support the technology.
Figure 8: A facility for receiving CO₂-carrying ships and injecting the CO₂ underground – under construction in Norway
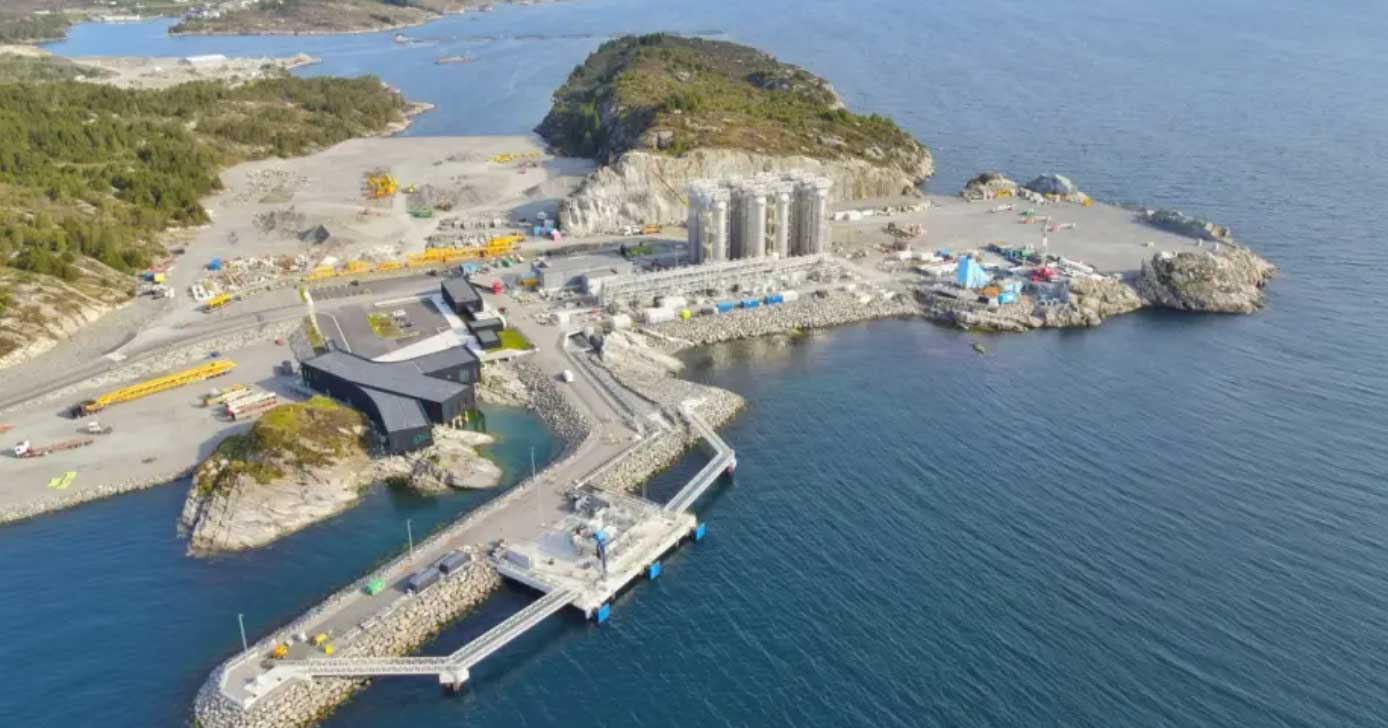
Is carbon capture and storage too expensive?
The cost of carbon capture and storage will depend heavily on the source of the CO₂, as more concentrated CO₂ costs less to capture. Current estimates suggest that lower-cost sources such as fertiliser plants and refineries could capture and store CO₂ for less than 90 euro per tonne, which is around the current cost of emitting CO₂ in the EU (in early 2022). Other industries, such as cement and waste-to-energy, may cost at least 120 euro per tonne of CO₂ stored, while direct air capture is currently estimated to cost between 350 and 700 euro per tonne.
For comparison, the early years of offshore wind development in the UK saw projects receive up to £70 per MWh over the average market price of electricity, which is equivalent to around €130 per tonne of CO₂ avoided from fossil-fired generation. Funding this technology has successfully driven down costs and today, no subsidy is required. Just as for offshore wind and other low-carbon technologies, it is expected that the cost of carbon capture and storage will fall as technologies are optimised, lenders become more comfortable with financing projects, and new projects can connect to already established infrastructure for transporting and storing CO₂.
Even at today’s costs, carbon capture and storage remains the lowest cost option for decarbonising many sources of CO₂, as well providing an indispensable service in permanently removing CO₂ from the atmosphere. This is why most detailed studies of possible ways to reach net zero show a significant role for carbon capture and storage in lowest cost solutions. A 2014 report from the IPCC indicated an average cost increase of 138% in pathways that excluded carbon capture and storage.
Reaching net zero will inevitably carry a cost for society, but one that will ultimately be less than the cost of dealing with the effects of global warming. Carbon capture and storage can help reduce that total cost.
Who will pay for carbon capture and storage?
Carbon-intensive industries will not capture and store their CO₂ emissions unless climate policy requires them to do so, while also ensuring they remain viable businesses. In Europe, the carbon price established by the Emissions Trading System is the principal tool for driving decarbonisation of large carbon emitters, but polluting industries are currently partly protected from paying this price so they can stay competitive with imported goods. In future, the carbon price may be high enough for industries to pay to capture and store CO₂, passing on the additional cost to customers in the price of low-carbon products. The cost of using carbon capture and storage to decarbonise raw materials, such as cement, steel, and plastics, can represent a relatively small increase in the cost of final products, such as buildings or cars (Figure 9). In future, governments and consumers may be able to absorb these costs as a premium for lowering their carbon footprint.
Figure 9: The relative price impact of decarbonised cement and steel in end-use products
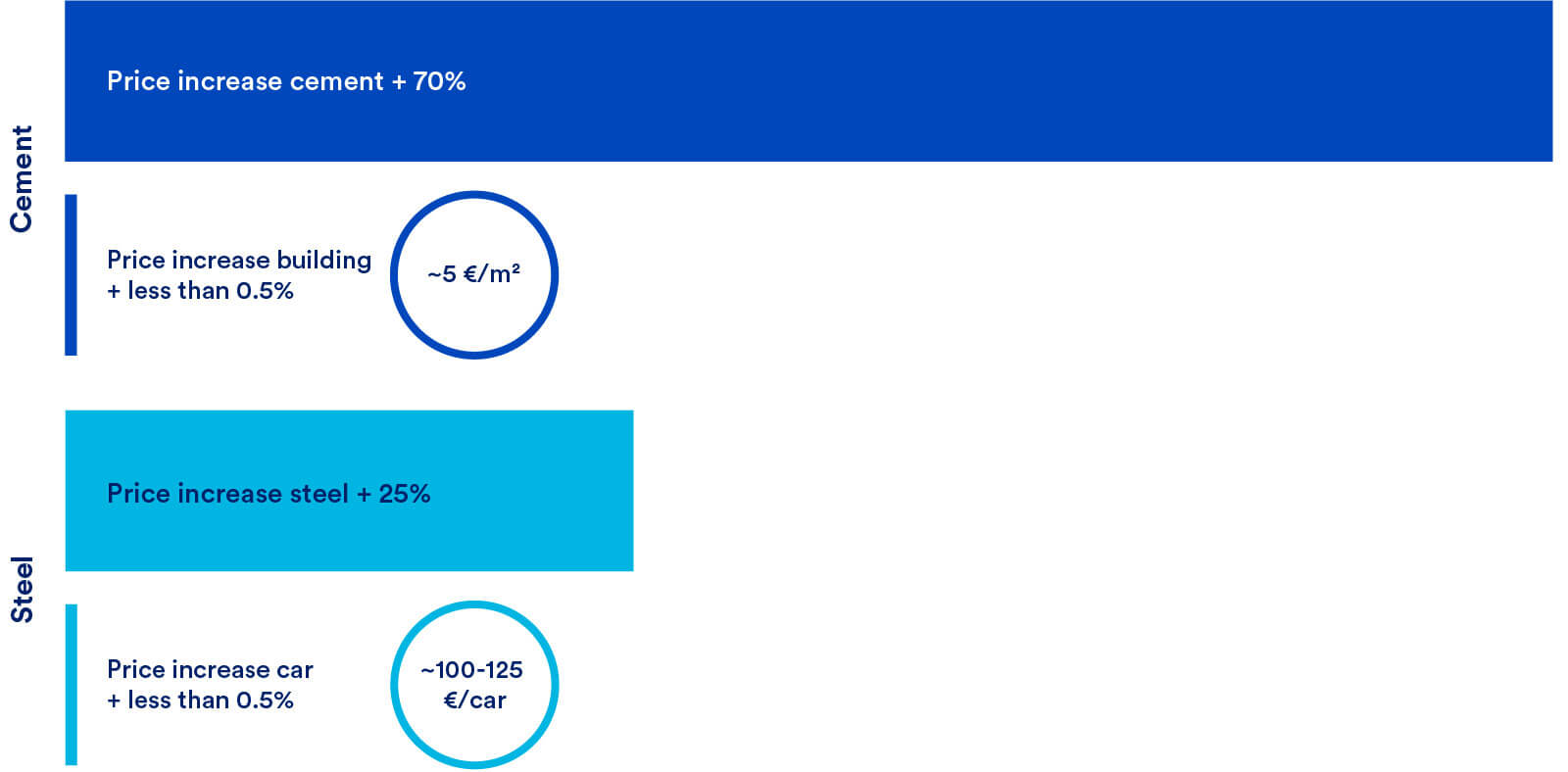
Most carbon capture and storage projects under development in Europe today face high costs as they bear the burden of new infrastructure development and use technologies that have yet to be mass-produced and optimised for cost. Governments can help bridge the financial gap faced by these early projects, particularly by funding ‘public good’ CO₂ transport infrastructure and storage sites that can be shared by many industries.
Policy can also help cover the shortfall in the carbon price by paying industries the difference between the cost of capturing and storing CO₂ and the cost of emitting. As the carbon price increases, governments pay less under this model. Using this approach, the Dutch government has guaranteed up to €2.1 billion for the large carbon capture and storage project planned in Rotterdam, but may not have to pay the projects at all if carbon prices remain above 2023 levels of over €80/t.
Government funding for the early development of carbon capture and storage can be likened to the support received by renewable energy, which has received annual subsidies in the EU surpassing €70 billion in the last five years. CATF analysis has shown that even the large funding allocations provided by the Norwegian and Dutch governments to launch carbon capture and storage projects represent less than average EU funding for wind and solar power over the past ten years, when compared on a basis of euros spent per tonne of CO₂ avoided.
What will be the impact on jobs and the economy?
Europe’s heavy industries directly employ nearly four million people, and are associated with many more indirect jobs. The steelmaking industry alone is estimated to be linked to 1.5 million more indirect jobs in the EU. Given that Europe will continue to be reliant on the products of these industries for the coming decades, these jobs and their benefit to local and national economies should be kept within the region, rather than lost to other parts of the world. Transforming carbon-intensive sectors using carbon capture and other technologies can also generate thousands of new, high-skilled jobs, such as those associated with new infrastructure for the transport and storage of CO₂. Perhaps most importantly, by taking a leading role on carbon capture and storage, Europe can develop new technologies, industries and expertise which can be exported to help drive the global transition to net zero.
What are the risks associated with carbon capture and storage?
Is there a risk of earthquakes caused by CO₂ storage?
CO₂ storage in saline reservoirs and depleted oil and gas reservoirs requires the controlled injection of the CO₂ into permeable rock formations. It is conducted under carefully controlled conditions to ensure the pressure in the formation does not reach levels that would fracture the rock or cause movement in geological fault lines, as this could compromise the site’s ability to contain CO₂. This is in contrast with many other commercial activities below ground, such as natural gas storage, extraction of oil and natural gas, or geothermal energy production, which carry a greater risk of inducing seismicity. In gas storage, the pressure is varied cyclically as gas is added or withdrawn, while gas production fields undergo a steady decline in the pressure. The injection of fluid into geothermal reservoirs has also been known to cause seismicity, but takes place under very different conditions to the injection of CO₂ into storage sites.
As a result, most recorded seismicity from CO₂ storage projects is of very low magnitude, below levels which can be felt by people. However, this will depend to a large extent on the geology of the site and the how the project is carried out. A review of seismicity induced by CO₂ storage projects notes only one project that has reported earthquakes of higher magnitudes, at the Cogdell enhanced oil recovery site in Texas.
Are there environmental risks associated with carbon capture and storage?
CO₂ is naturally present in the air and is produced by all living things. It is not harmful at low concentrations, but at sufficiently high concentrations it can displace oxygen in the air and asphyxiate people and animals. Increasing CO₂ concentrations can stimulate plant growth, but will eventually also cause plant death from lack of oxygen.
Although CO₂ storage sites are highly unlikely to release CO₂, various research projects have performed controlled releases of CO₂ to examine the possible effects were any CO₂ to be emitted from a storage site. Simulation of a gradual leak from the seabed by the GEOMAR Helmholtz Centre for Ocean Research showed that CO₂ would rapidly dissolve in seawater, with a very localised impact on marine life due to acidification. Simulation of an underground CO₂ release on land by Montana State University has similarly been shown to have a very localised impact on plant life (around 2.5 metres from the leak), due to elevated CO₂ concentrations in the soil.
When assessing the environmental and health risks of carbon capture and storage, it is important to also consider the risks of not using carbon capture and storage. Climate change has already caused significant environmental damage, which will only worsen so long as CO₂ produced by humans continues to pollute the atmosphere. Carbon capture and storage can help slow and prevent this continued carbon pollution, reducing the environmental damage caused by climate change.
Are there risks to human health with carbon capture and storage?
CO₂ sinks in air, so can potentially accumulate in low-lying areas and become an asphyxiation risk. In volcanic regions such as parts of Italy, humans have lived for millennia alongside natural CO₂ vents, most of which produce CO₂ at rates far greater (up to 100 tonnes per day) than would be expected from failure of a manmade storage site. These have very occasionally caused fatalities, particularly when people have remained close to the ground in geographical dips. However, research by the University of Edinburgh have shown that the risk posed even by these vents is lower than the risk of lightning strike, or the chances of winning the lottery.
There is also a risk of exposure to CO₂ from accidental releases during transportation, for instance, from a pipeline, truck, or storage tank, if large volumes of CO₂ were allowed to accumulate in a confined area. CO₂ is already safely transported by rail, road and ship for commercial uses such as the food and beverage industry, and in the Netherlands, there is a large network of pipelines supplying industrial CO₂ to greenhouses, with no reported injuries or deaths. In the USA, CO₂ has been transported for 50 years for oil field operations, using a pipeline network which is now 8000 km in length, with one recorded injury and no deaths. Most leakage incidents have released less than 10 tonnes of CO₂. However, a rare large pipeline rupture caused by a landslide in Satartia, Mississippi in 2020 led to 45 people seeking medical attention.
Are there alternatives to carbon capture and storage?
Why can’t we decarbonise using cheap wind and solar power?
Consistent policy support for wind and solar power has allowed them to become highly effective, low-cost options for decarbonising power generation in Europe and the rest of the world. However, low-carbon electricity can only help decarbonise the parts of our economy that can be electrified. Heavy industries such as the production of cement, steel, and chemicals rely on many processes that are challenging or impossible to replace with electricity. Cement and some chemicals release CO₂, not from fossil fuel combustion, but from the chemical reactions fundamental to their production. Other processes require high temperatures that are very difficult to produce using electricity alone.
Even if we are able to use renewable electricity to address an ever-increasing proportion of our emissions, the challenge is so great that we need to use all the tools we have available today. In Europe, only around 38% of electricity generation is renewable – carbon capture and storage can help reduce the demand for low-carbon electricity and prioritise it for uses where it is most needed, such as heating homes and powering electric transport.
Will we still need heavy industry in a low-carbon world?
Minimising our reliance on manufactured goods through lower consumption levels and increased recycling can play a vital role in reducing emissions from heavy industry. Historically, economies have become increasingly material-intensive as they have developed, although some developed countries are showing signs of breaking this dependency. Demand for cement, steel, and chemicals in Europe is nevertheless projected to remain fairly constant to 2050, even as recycling rates are increased. The rapid growth of the renewable industry is just one example of how these fundamental materials will continue to be needed in future. The EU’s growth in wind and solar power could require over 8 million tonnes of annual steel production by 2050
Using CO₂ storage for carbon dioxide removal
How can carbon capture and storage technologies be used for carbon dioxide removal?
As well as helping to reduce our CO₂ emissions, carbon capture and storage technologies can be used to permanently remove the greenhouse gas from the atmosphere – a process which is also known as ‘carbon dioxide removal’ or CDR. Direct air capture and storage (DACS) uses CO₂-binding chemicals or materials to separate the greenhouse gas from the air, before permanently storing the captured CO₂ in deep rock formations. CO₂ removal can also be achieved by capturing and storing the CO₂ released when biomass is burned for power or converted into other fuels. For these technologies to truly remove CO₂ from the atmosphere, it is vital to account for all the uncaptured emissions associated with the processes and ensure that any biomass used benefits both the climate and ecosystems. Although they play distinct roles in reaching our climate goals, the permanent storage of atmospheric and fossil CO₂ share many of the same challenges, particularly regarding the development of CO₂ transport and storage infrastructure.
Why should we remove CO₂ from the atmosphere as well as reduce our emissions?
Reductions in greenhouse gas emissions should always be prioritised over removal of CO₂ from the atmosphere, as permanent removal is usually more costly and – if done too late – risks effects on the climate that could be difficult to reverse. However, emissions from some sectors, such as aviation and shipping, may be impossible to eliminate by 2050 or will cost less to remove from the atmosphere. Some removal will therefore be necessary to balance these ‘residual emissions’ at net zero. In addition, the European Climate Law includes a commitment for the EU to go ‘net negative’ after 2050, meaning more CO₂ will need to be removed from the atmosphere than is emitted. In recognition of these ambitions, the EU and many national governments are making growing efforts to expand a range of CO₂ removal technologies, including direct air capture and bioenergy with carbon capture and storage. The need for these kinds of CO₂ removal technologies are also clearly highlighted by the latest assessment by the International Panel on Climate Change, in which carbon removal using geological storage makes a significant contribution in over 90% of scenarios that limit warming to 1.5°C.