Bioenergia benefica per il clima: Opportunità e sfide
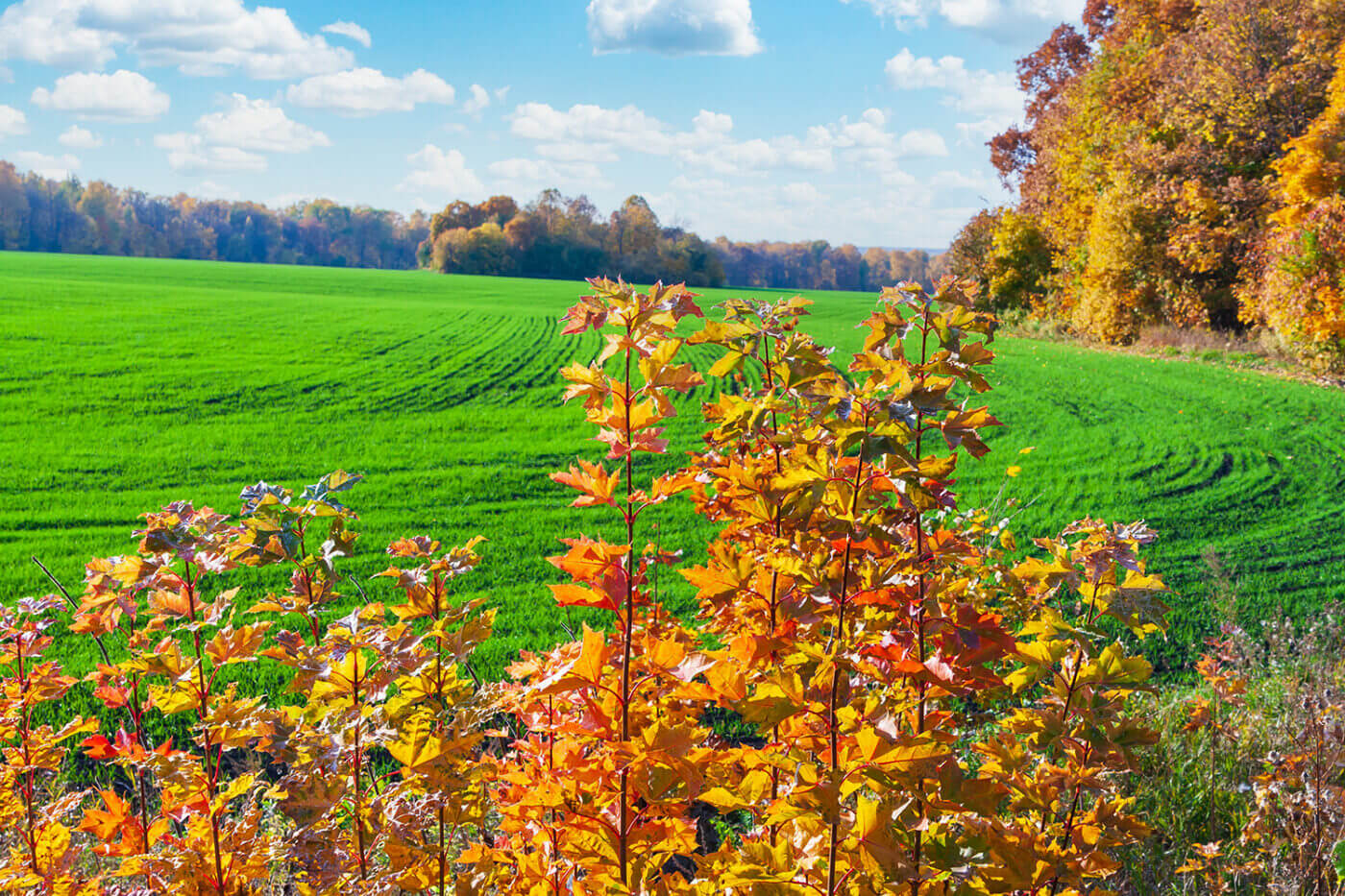
What is climate-beneficial bioenergy?
For bioenergy systems to be climate-beneficial they must couple biomass resources with engineered processes and technologies to reduce greenhouse gas emissions and draw down atmospheric carbon dioxide for permanent storage. This will result in usable energy and coproducts alongside sustaining agriculture and Ecosystem Carbon Management which is the intentional management of ecosystems to enhance and maintain carbon storage.
“Climate-Beneficial Bioenergy,” a concept developed by CATF, aims to advance modern bioenergy systems that satisfy certain requirements to ensure positive climate outcomes. Even as we define and advance climate-beneficial systems it is critical to continue to safeguard against detrimental impacts of conventional bioenergy systems. While a majority of current bioenergy systems do not yet meet the high bar of “climate-beneficial” systems, commercial examples are emerging, and establishing key criteria or definitions for what constitutes a climate-beneficial system can help drive improvement.
Why are climate-beneficial bioenergy systems important?
Climate-beneficial bioenergy systems could play an important role in sectors that require local, firm, dispatchable power or carbon dioxide removal (CDR) to address emissions that are technically or economically difficult to reduce. The carbon in biomass resources can be used as a replacement carbon source in systems otherwise reliant on fossil fuels. Through enhanced photosynthesis, with proper management, biomass systems can also draw down atmospheric carbon dioxide concentrations as carbon dioxide removal (CDR).
The opportunity for utilizing biomass resources to achieve climate benefits stems from their ability to capture dilute atmospheric carbon dioxide via photosynthesis and concentrate it for permanent storage.1 Economic sectors like agriculture that are expected to have emissions that cannot be addressed technically or economically may require biomass-based carbon dioxide removal technologies to counterbalance these residual emissions. While all biomass-based carbon dioxide removal systems divert gross carbon atoms to storage, a lifecycle assessment is needed to understand whether a particular bioenergy system constitutes a net greenhouse gas emission, emissions reduction, or carbon dioxide removal.
Given that biomass can be a food source, cleans the air and water, and stores carbon, the use of biomass resources has the potential to enhance or maintain ecosystem carbon sinks and support services such as soil health, water quality, food security, rural economic development, health, air quality, energy, and social equity. When not managed properly, however, utilizing biomass resources can risk these services and undermine efforts to reduce land competition and preserve existing carbon sinks.
It’s essential that bioenergy systems and policies are designed to ensure overall climate benefit. Bioenergy technologies and policies should focus on biomass resources that are compatible with food production, avoid deforestation, maintain and enhance land carbon sinks, protect air quality, support multi-use landscapes that benefit ecosystems and communities, and enhance circularity in agricultural systems by utilizing waste streams and reducing the need for new system inputs. They preferably direct bioenergy to hard-to-decarbonize sectors, catalyze carbon capture and storage at new and existing bioenergy facilities, and advance performance-based policies that achieve environmental and climate-beneficial outcomes.
Examples of climate-beneficial bioenergy system attributes:
- Capture carbon dioxide from the atmosphere through photosynthesis and transfer carbon atoms to permanent storage.
- Recover biomass resources that would otherwise emit greenhouse gases.
- Are coupled with improved agricultural and forest management that supports biodiversity, ecosystem resilience, communities2, sustainable intensification of productive farmland and multi-use landscapes (food and bioenergy),3 and enhance circularity in agriculture by utilizing wastes and reducing system inputs.4
- Produce coproducts that substitute fossil-derived products and reduce greenhouse gas emissions and other losses like nitrate-nitrogen.
- Provide local, firm, dispatchable power that emits lower greenhouse gas emissions than the alternative energy source and/or alternative scenario for the biomass resource.
- Provide energy and other products for hard-to-decarbonize sectors.
- Achieve net carbon dioxide removal.
What are the different approaches to climate-beneficial bioenergy?
When determining whether a bioenergy system is climate-beneficial, it is important to assess the biomass resources used as well as the conversion processes and technologies (see Box 1). The table below provides examples of these two features for both conventional bioenergy systems and those that have the potential to be climate-beneficial.
Biomass resources | Conversion processes and technologies |
---|---|
Examples of conventional bioenergy | |
Examples of climate-beneficial bioenergy | |
Box 1: Key terminology
Biomass resources
Biomass resources are organic materials from the biosphere, including woody and herbaceous plants, manure, and organic municipal waste.8 Unlike fossil fuels that are formed over geologic timeframes, biomass resources originate from recent biological activity. Plant biomass is a valuable natural resource that provides food, cleans air and water, and stores carbon dioxide. Humans and other living things consume plants to harness this chemical energy but also excrete some biomass resources in their wastes, such as manure. Biomass resources such as perennial grasses and crop residues, forest thinnings, food processing waste, and construction debris can be managed and sourced to produce climate-beneficial bioenergy.
Conversion processes and technologies
Bioenergy conversion processes and technologies use stored chemical energy and other elements in biomass resources to produce bioenergy. Different carbon capture and storage technologies can be combined with conversion processes and technologies to transfer and permanently store some of the carbon atoms released during bioenergy conversion processes. These systems achieve net carbon dioxide removal when the amount of carbon atoms permanently stored is greater than the carbon dioxide-equivalent amount of greenhouse gas emitted throughout the system’s supply chain over its lifecycle. Bioenergy conversion processes and technologies also produce coproducts, such as nitrogen-rich liquid digestate, that can be used as a synthetic fertilizer replacement, or biocoke, which can substitute coal in emissions-intensive processes such as steel manufacturing.
Advancing climate-beneficial bioenergy
As interest in climate-beneficial bioenergy systems continues to emerge, appropriate policy structures must be developed to advance both financial incentives for implementation and safeguards to ensure these approaches have real climate benefits and do not detrimentally affect people or the environment. Adopting “climate-beneficial bioenergy” as a concept will encourage innovation in modern approaches to harnessing photosynthesis and using land systems, approaches that have emerged since the rapid scale-up of conventional bioenergy systems linked to detrimental effects. Identifying modern climate-beneficial bioenergy systems and their appropriate uses while safeguarding conventional detrimental bioenergy systems is needed to ensure positive climate outcomes for both people and the environment.
Note a piè di pagina
- Richard (2021). https://sdg-action.org/harnessing-the-power-of-photosynthesis-for-negative-emissions/
- Schulte et al. (2022) Meeting global challenges with regenerative agriculture producing food and energy. Nature Sustainability. https://doi.org/10.1038/s41893-021-00827-y
- Malone et al. (2023) Harvested winter rye energy cover crop: multiple benefits for North Central US. Environmental Research Letters. DOI 10.1088/1748-9326/acd708
- Lower et al. (2022) Coupling Circularity with Carbon Negativity in Food and Agricultural Systems. Journal of ASABE. https://doi.org/10.13031/ja.14908
- “Transesterification describes the process by which triglycerides are reacted with methanol or ethanol to produce methyl esters and ethyl esters, respectively, along with the coproduct glycerol.” Brown, R. C., & Brown, T. R. (2013). Biorenewable resources: engineering new products from agriculture. John Wiley & Sons.
- The term “forest thinnings” is used here to refer to trees that are removed to improve forest health and reduce wildfire risk.
- Socially marginal land refers to land that is earning close to zero returns after accounting for the monetized costs of environmental externalities generated under crop production as defined by Khanna et al. (2021) Redefining marginal land for bioenergy crop production. GCB Bioenergy. https://doi.org/10.1111/gcbb.12877
- Brown, R. C., & Brown, T. R. (2013). Biorenewable resources: engineering new products from agriculture. John Wiley & Sons.
Learn more about CATF’s leading work on climate-beneficial bioenergy; contact us at [email protected].